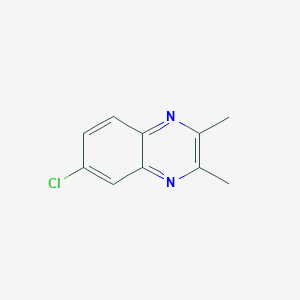
6-Chloro-2,3-dimethylquinoxaline
Overview
Description
Dibutyltin dilaurate is an organotin compound with the chemical formula (C₄H₉)₂Sn(O₂C₁₂H₂₃)₂. It is a colorless to yellowish, viscous, and oily liquid. This compound is widely used as a catalyst in various industrial processes, including the production of polyurethanes, silicones, and other polymers .
Preparation Methods
Synthetic Routes and Reaction Conditions: Dibutyltin dilaurate is typically synthesized by reacting dibutyltin oxide with lauric acid. The reaction involves heating the mixture to a temperature between 80 and 88°C under negative pressure for about 2 hours. Acetic anhydride is then added, and the reaction continues for an additional hour with stirring .
Industrial Production Methods: In industrial settings, the preparation of dibutyltin dilaurate follows a similar process but on a larger scale. The reaction conditions are optimized to ensure high yield and purity of the product. The use of advanced equipment and controlled environments helps in achieving consistent quality .
Chemical Reactions Analysis
Types of Reactions: Dibutyltin dilaurate undergoes various chemical reactions, including:
Esterification: It catalyzes the formation of esters from carboxylic acids and alcohols.
Transesterification: It facilitates the exchange of ester groups between molecules.
Polycondensation: It aids in the formation of polymers by linking monomer units.
Common Reagents and Conditions:
Esterification and Transesterification: These reactions typically occur in the presence of alcohols and carboxylic acids at elevated temperatures.
Polycondensation: This reaction involves monomers with reactive end groups, such as hydroxyl or carboxyl groups, under controlled temperature and pressure.
Major Products:
Polyurethanes: Formed from the reaction of isocyanates and polyols.
Silicones: Produced through the vulcanization of siloxanes.
Scientific Research Applications
Dibutyltin dilaurate has a wide range of applications in scientific research:
Chemistry: It is used as a catalyst in the synthesis of various polymers and organic compounds.
Medicine: Research has explored its potential in drug delivery systems and as a component in biomaterials.
Industry: It is extensively used in the production of coatings, adhesives, sealants, and elastomers.
Mechanism of Action
Dibutyltin dilaurate acts as a catalyst by facilitating the formation of urethane bonds through the reaction of isocyanates with alcohols. The tin atom in the compound coordinates with the reactants, lowering the activation energy and accelerating the reaction. This mechanism is particularly effective for both aliphatic and aromatic isocyanates .
Comparison with Similar Compounds
Dibutyltin dioctanoate: Another organotin compound used as a catalyst in similar applications.
Dibutyltin diacetate: Used in esterification and transesterification reactions.
Uniqueness: Dibutyltin dilaurate is unique due to its high catalytic activity and versatility in various industrial processes. Its ability to catalyze multiple types of reactions, including esterification, transesterification, and polycondensation, makes it a valuable compound in both research and industrial applications .
Properties
IUPAC Name |
6-chloro-2,3-dimethylquinoxaline | |
---|---|---|
Source | PubChem | |
URL | https://pubchem.ncbi.nlm.nih.gov | |
Description | Data deposited in or computed by PubChem | |
InChI |
InChI=1S/C10H9ClN2/c1-6-7(2)13-10-5-8(11)3-4-9(10)12-6/h3-5H,1-2H3 | |
Source | PubChem | |
URL | https://pubchem.ncbi.nlm.nih.gov | |
Description | Data deposited in or computed by PubChem | |
InChI Key |
CNNSWSHYGANWBM-UHFFFAOYSA-N | |
Source | PubChem | |
URL | https://pubchem.ncbi.nlm.nih.gov | |
Description | Data deposited in or computed by PubChem | |
Canonical SMILES |
CC1=C(N=C2C=C(C=CC2=N1)Cl)C | |
Source | PubChem | |
URL | https://pubchem.ncbi.nlm.nih.gov | |
Description | Data deposited in or computed by PubChem | |
Molecular Formula |
C10H9ClN2 | |
Source | PubChem | |
URL | https://pubchem.ncbi.nlm.nih.gov | |
Description | Data deposited in or computed by PubChem | |
DSSTOX Substance ID |
DTXSID90284716 | |
Record name | 6-Chloro-2,3-dimethylquinoxaline | |
Source | EPA DSSTox | |
URL | https://comptox.epa.gov/dashboard/DTXSID90284716 | |
Description | DSSTox provides a high quality public chemistry resource for supporting improved predictive toxicology. | |
Molecular Weight |
192.64 g/mol | |
Source | PubChem | |
URL | https://pubchem.ncbi.nlm.nih.gov | |
Description | Data deposited in or computed by PubChem | |
Solubility |
9.4 [ug/mL] (The mean of the results at pH 7.4) | |
Record name | SID26728807 | |
Source | Burnham Center for Chemical Genomics | |
URL | https://pubchem.ncbi.nlm.nih.gov/bioassay/1996#section=Data-Table | |
Description | Aqueous solubility in buffer at pH 7.4 | |
CAS No. |
17911-93-2 | |
Record name | NSC38597 | |
Source | DTP/NCI | |
URL | https://dtp.cancer.gov/dtpstandard/servlet/dwindex?searchtype=NSC&outputformat=html&searchlist=38597 | |
Description | The NCI Development Therapeutics Program (DTP) provides services and resources to the academic and private-sector research communities worldwide to facilitate the discovery and development of new cancer therapeutic agents. | |
Explanation | Unless otherwise indicated, all text within NCI products is free of copyright and may be reused without our permission. Credit the National Cancer Institute as the source. | |
Record name | 6-Chloro-2,3-dimethylquinoxaline | |
Source | EPA DSSTox | |
URL | https://comptox.epa.gov/dashboard/DTXSID90284716 | |
Description | DSSTox provides a high quality public chemistry resource for supporting improved predictive toxicology. | |
Retrosynthesis Analysis
AI-Powered Synthesis Planning: Our tool employs the Template_relevance Pistachio, Template_relevance Bkms_metabolic, Template_relevance Pistachio_ringbreaker, Template_relevance Reaxys, Template_relevance Reaxys_biocatalysis model, leveraging a vast database of chemical reactions to predict feasible synthetic routes.
One-Step Synthesis Focus: Specifically designed for one-step synthesis, it provides concise and direct routes for your target compounds, streamlining the synthesis process.
Accurate Predictions: Utilizing the extensive PISTACHIO, BKMS_METABOLIC, PISTACHIO_RINGBREAKER, REAXYS, REAXYS_BIOCATALYSIS database, our tool offers high-accuracy predictions, reflecting the latest in chemical research and data.
Strategy Settings
Precursor scoring | Relevance Heuristic |
---|---|
Min. plausibility | 0.01 |
Model | Template_relevance |
Template Set | Pistachio/Bkms_metabolic/Pistachio_ringbreaker/Reaxys/Reaxys_biocatalysis |
Top-N result to add to graph | 6 |
Feasible Synthetic Routes
Q1: What is the primary focus of the research article on 6-Chloro-2,3-dimethylquinoxaline 1,4-dioxide?
A1: The research article primarily focuses on experimentally determining the thermochemical properties of this compound 1,4-dioxide. [] This includes measuring the enthalpy of formation for the compound. Additionally, the research utilizes Density Functional Theory (DFT) calculations to evaluate the N–O bond enthalpies in related haloquinoxalines, providing insights into the influence of halogen substituents on bond strength. []
Q2: Why is understanding the N–O bond enthalpy important in the context of haloquinoxalines?
A2: Understanding the N–O bond enthalpy in haloquinoxalines is crucial for predicting their reactivity and potential applications. [] The strength of this bond can significantly influence the compound's stability and its ability to participate in chemical reactions. By studying the impact of halogen substituents on N–O bond enthalpy, researchers can gain valuable insights into structure-property relationships within this class of compounds.
Disclaimer and Information on In-Vitro Research Products
Please be aware that all articles and product information presented on BenchChem are intended solely for informational purposes. The products available for purchase on BenchChem are specifically designed for in-vitro studies, which are conducted outside of living organisms. In-vitro studies, derived from the Latin term "in glass," involve experiments performed in controlled laboratory settings using cells or tissues. It is important to note that these products are not categorized as medicines or drugs, and they have not received approval from the FDA for the prevention, treatment, or cure of any medical condition, ailment, or disease. We must emphasize that any form of bodily introduction of these products into humans or animals is strictly prohibited by law. It is essential to adhere to these guidelines to ensure compliance with legal and ethical standards in research and experimentation.