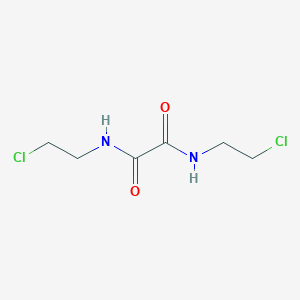
N,N'-Bis(2-chloroethyl)oxamide
Overview
Description
N,N’-Bis(2-chloroethyl)oxamide, also known as HN2, is a synthetic nitrogen-containing compound with a variety of applications in scientific research. It is used as a reagent in organic synthesis, as a tool for studying the effects of alkylating agents on DNA, and as a tool for studying the effects of alkylating agents on proteins. HN2 has been used in the development of novel pharmaceuticals, in the study of the effects of radiation on biological systems, and in the study of the effects of environmental toxins.
Scientific Research Applications
Coordination Chemistry : N,N'-Bis(coordinating group substituted)oxamides are used to design homo- and heterometallic species due to their easy cis-trans isomerization equilibria and the variety of substituents that can alter charge, complexing ability, and polarity. They are effective in stabilizing high oxidation states of late first-row transition metal ions and show potential in mediating antiferromagnetic interactions between paramagnetic centers (Ruiz et al., 1999).
Structural Investigation : N,N'-Bis(2-hydroxyphenyl)oxamide and its derivatives demonstrate intramolecular three-center hydrogen bonding. Their structures are confirmed through NMR and X-ray diffraction studies, showing planar conformation and trans configuration in solid state, which is relevant for understanding their chemical behavior (Martínez-Martínez et al., 1998).
Bimetallic Complexes : Asymmetric N,N'-Bis(substituted)oxamide ligands are used to synthesize bimetallic complexes with potential in vitro cytotoxic activities and reactivities towards DNA and protein. This highlights their potential application in medicinal chemistry and cancer research (Li et al., 2012).
Spectrophotometric Studies : N,N'-Bis(2-Sulphoethyl)dithio-oxamide has been used as a reagent for palladium, forming water-soluble chelates with palladium(II) in acid solutions. This application is significant in analytical chemistry for the detection and quantification of palladium (Goeminne et al., 1963).
NMR Study of Oxamide Derivatives : The conformational behavior of various oxamide derivatives, including N,N'-Bis[(2-hydroxy)phenyl]oxamide, has been studied using NMR spectroscopy. This provides insights into their structural and electronic properties (Martínez-Martínez et al., 1993).
Cancer Research : N,N'-Bis(2-chloroethyl)oxamide derivatives, as metabolites of the antitumor agent cyclophosphamide, have been identified and studied for their cytotoxic properties, contributing to our understanding of chemotherapy drug metabolism and action (Struck et al., 1971).
Polyurethane Foam Production : N,N'-Bis(2-hydroxyethyl)oxamide derivatives have been used in the synthesis of polyurethane foams, demonstrating enhanced thermal stability and mechanical properties. This application is relevant in materials science and engineering (Zarzyka et al., 2012).
Safety and Hazards
This compound may decompose upon combustion or in high temperatures to generate poisonous fumes . In case of contact, it is advised to remove the victim to fresh air and keep at rest in a position comfortable for breathing . If skin irritation or rash occurs, or if eye irritation persists after rinsing, it is recommended to get medical advice/attention .
Properties
IUPAC Name |
N,N'-bis(2-chloroethyl)oxamide | |
---|---|---|
Source | PubChem | |
URL | https://pubchem.ncbi.nlm.nih.gov | |
Description | Data deposited in or computed by PubChem | |
InChI |
InChI=1S/C6H10Cl2N2O2/c7-1-3-9-5(11)6(12)10-4-2-8/h1-4H2,(H,9,11)(H,10,12) | |
Source | PubChem | |
URL | https://pubchem.ncbi.nlm.nih.gov | |
Description | Data deposited in or computed by PubChem | |
InChI Key |
MUAULJMMFLVLHM-UHFFFAOYSA-N | |
Source | PubChem | |
URL | https://pubchem.ncbi.nlm.nih.gov | |
Description | Data deposited in or computed by PubChem | |
Canonical SMILES |
C(CCl)NC(=O)C(=O)NCCCl | |
Source | PubChem | |
URL | https://pubchem.ncbi.nlm.nih.gov | |
Description | Data deposited in or computed by PubChem | |
Molecular Formula |
C6H10Cl2N2O2 | |
Source | PubChem | |
URL | https://pubchem.ncbi.nlm.nih.gov | |
Description | Data deposited in or computed by PubChem | |
DSSTOX Substance ID |
DTXSID40292568 | |
Record name | N,N'-Bis(2-chloroethyl)oxamide | |
Source | EPA DSSTox | |
URL | https://comptox.epa.gov/dashboard/DTXSID40292568 | |
Description | DSSTox provides a high quality public chemistry resource for supporting improved predictive toxicology. | |
Molecular Weight |
213.06 g/mol | |
Source | PubChem | |
URL | https://pubchem.ncbi.nlm.nih.gov | |
Description | Data deposited in or computed by PubChem | |
CAS No. |
16813-43-7 | |
Record name | 16813-43-7 | |
Source | DTP/NCI | |
URL | https://dtp.cancer.gov/dtpstandard/servlet/dwindex?searchtype=NSC&outputformat=html&searchlist=83698 | |
Description | The NCI Development Therapeutics Program (DTP) provides services and resources to the academic and private-sector research communities worldwide to facilitate the discovery and development of new cancer therapeutic agents. | |
Explanation | Unless otherwise indicated, all text within NCI products is free of copyright and may be reused without our permission. Credit the National Cancer Institute as the source. | |
Record name | N,N'-Bis(2-chloroethyl)oxamide | |
Source | EPA DSSTox | |
URL | https://comptox.epa.gov/dashboard/DTXSID40292568 | |
Description | DSSTox provides a high quality public chemistry resource for supporting improved predictive toxicology. | |
Retrosynthesis Analysis
AI-Powered Synthesis Planning: Our tool employs the Template_relevance Pistachio, Template_relevance Bkms_metabolic, Template_relevance Pistachio_ringbreaker, Template_relevance Reaxys, Template_relevance Reaxys_biocatalysis model, leveraging a vast database of chemical reactions to predict feasible synthetic routes.
One-Step Synthesis Focus: Specifically designed for one-step synthesis, it provides concise and direct routes for your target compounds, streamlining the synthesis process.
Accurate Predictions: Utilizing the extensive PISTACHIO, BKMS_METABOLIC, PISTACHIO_RINGBREAKER, REAXYS, REAXYS_BIOCATALYSIS database, our tool offers high-accuracy predictions, reflecting the latest in chemical research and data.
Strategy Settings
Precursor scoring | Relevance Heuristic |
---|---|
Min. plausibility | 0.01 |
Model | Template_relevance |
Template Set | Pistachio/Bkms_metabolic/Pistachio_ringbreaker/Reaxys/Reaxys_biocatalysis |
Top-N result to add to graph | 6 |
Feasible Synthetic Routes
Disclaimer and Information on In-Vitro Research Products
Please be aware that all articles and product information presented on BenchChem are intended solely for informational purposes. The products available for purchase on BenchChem are specifically designed for in-vitro studies, which are conducted outside of living organisms. In-vitro studies, derived from the Latin term "in glass," involve experiments performed in controlled laboratory settings using cells or tissues. It is important to note that these products are not categorized as medicines or drugs, and they have not received approval from the FDA for the prevention, treatment, or cure of any medical condition, ailment, or disease. We must emphasize that any form of bodily introduction of these products into humans or animals is strictly prohibited by law. It is essential to adhere to these guidelines to ensure compliance with legal and ethical standards in research and experimentation.