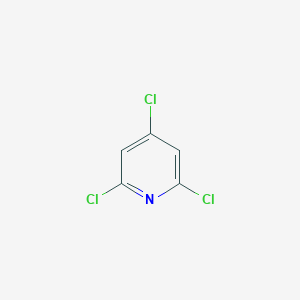
2,4,6-Trichloropyridine
Overview
Description
2,4,6-Trichloropyridine is an organic compound with the molecular formula C5H2Cl3N. It is a derivative of pyridine, where three hydrogen atoms are replaced by chlorine atoms at the 2, 4, and 6 positions. This compound is known for its crystalline powder form, which ranges in color from yellow to yellow-brown . It has a melting point of 33-37°C and a boiling point of 217.5-218.5°C .
Mechanism of Action
Target of Action
2,4,6-Trichloropyridine is a biochemical reagent that can be used as a biological material or organic compound for life science related research . .
Biochemical Pathways
It is known that pyridine derivatives can influence various biochemical pathways, depending on their specific targets .
Result of Action
It is known that pyridine derivatives can have various effects at the molecular and cellular level, depending on their specific targets .
Action Environment
The action, efficacy, and stability of this compound can be influenced by various environmental factors. These may include the pH of the environment, the presence of other substances, and temperature .
Biochemical Analysis
Biochemical Properties
2,4,6-Trichloropyridine has been found to have a high reactivity, which allows it to react with compounds containing thiol groups to form disulfide bonds . This property allows it to bind multiple drugs to a single polymer, which can then be released simultaneously within the cytoplasm of cells, thereby improving the overall efficiency of treatment .
Cellular Effects
It is known that the compound can have different fluorescence response effects under different pH and solvent conditions . This property could potentially be utilized in optical sensing applications .
Preparation Methods
Synthetic Routes and Reaction Conditions: 2,4,6-Trichloropyridine can be synthesized from 2,6-dichloropyridine N-oxide. The process involves the use of hydrogen peroxide and trifluoroacetic acid as reagents . Another method involves the chlorination of 2,6-dichloropyridine using phosphorus oxychloride .
Industrial Production Methods: In industrial settings, this compound is typically produced by reacting barbituric acid with phosphorus oxychloride in the presence of a deacidification agent . This method is preferred due to its high yield and reduced environmental impact compared to other methods.
Chemical Reactions Analysis
Types of Reactions: 2,4,6-Trichloropyridine undergoes various chemical reactions, including:
Substitution Reactions: It can react with nucleophiles, such as amines, to form substituted pyridines.
Oxidation and Reduction: Although less common, it can participate in oxidation and reduction reactions under specific conditions.
Common Reagents and Conditions:
Substitution Reactions: Typically involve reagents like amines and catalysts such as copper iodide and palladium complexes.
Oxidation and Reduction: These reactions may require strong oxidizing or reducing agents, depending on the desired product.
Major Products:
Substitution Reactions: Products include various substituted pyridines, depending on the nucleophile used.
Oxidation and Reduction: Products vary based on the specific reaction conditions and reagents.
Scientific Research Applications
2,4,6-Trichloropyridine has a wide range of applications in scientific research:
Chemistry: It is used as an intermediate in the synthesis of various organic compounds.
Biology: It is utilized in the development of biologically active molecules and pharmaceuticals.
Medicine: It serves as a precursor for the synthesis of drugs and other therapeutic agents.
Industry: It is employed in the production of agrochemicals, dyes, and other industrial chemicals.
Comparison with Similar Compounds
- 2,6-Dichloropyridine
- 2,4,6-Trichloropyrimidine
- 2,3,6-Trichloropyridine
- 2,4,6-Trichloronicotinic acid
Comparison: 2,4,6-Trichloropyridine is unique due to its specific substitution pattern, which imparts distinct reactivity and properties compared to other chloropyridines. For instance, 2,6-dichloropyridine lacks the third chlorine atom, resulting in different reactivity and applications . Similarly, 2,4,6-Trichloropyrimidine, while structurally similar, has different chemical properties due to the presence of a nitrogen atom in the ring .
Biological Activity
2,4,6-Trichloropyridine (TCP) is a chlorinated derivative of pyridine with significant biological activity. Its molecular formula is and it has a molecular weight of 182.44 g/mol. TCP is primarily utilized in agricultural applications as a herbicide and fungicide, but its biological effects extend beyond these uses. This article reviews the biological activity of TCP, focusing on its pharmacological properties, toxicological effects, and potential applications in various fields.
Property | Value |
---|---|
Molecular Formula | |
Molecular Weight | 182.44 g/mol |
Melting Point | 33-37 °C |
Boiling Point | 212.5 °C |
Density | 1.5 g/cm³ |
Solubility in Water | Sparingly soluble |
Pharmacological Activity
TCP exhibits various pharmacological activities that can be categorized into several areas:
Antimicrobial Activity
TCP has demonstrated antimicrobial properties against a range of pathogens. Studies indicate that TCP can inhibit the growth of certain bacteria and fungi, making it a candidate for use in agricultural and medical applications.
- Case Study : A study published in the Journal of Agricultural and Food Chemistry found that TCP effectively reduced fungal growth in crops, suggesting its utility as a fungicide .
Insecticidal Properties
TCP is known for its insecticidal activity, particularly against pests that affect agricultural yields.
- Research Findings : Research has shown that TCP acts as an effective insecticide against various agricultural pests, including aphids and beetles, by disrupting their physiological processes .
Toxicological Effects
Despite its beneficial uses, TCP poses certain health risks due to its toxicity:
Acute Toxicity
TCP is classified as harmful if ingested or inhaled. It can cause skin and eye irritation upon contact.
- Toxicity Studies : Animal studies have indicated that TCP can lead to liver damage and other systemic effects when administered at high doses . The LD50 (lethal dose for 50% of the population) values suggest significant toxicity levels comparable to other chlorinated compounds.
Environmental Impact
The environmental persistence of TCP raises concerns regarding its accumulation in ecosystems:
Properties
IUPAC Name |
2,4,6-trichloropyridine | |
---|---|---|
Source | PubChem | |
URL | https://pubchem.ncbi.nlm.nih.gov | |
Description | Data deposited in or computed by PubChem | |
InChI |
InChI=1S/C5H2Cl3N/c6-3-1-4(7)9-5(8)2-3/h1-2H | |
Source | PubChem | |
URL | https://pubchem.ncbi.nlm.nih.gov | |
Description | Data deposited in or computed by PubChem | |
InChI Key |
FJNNGKMAGDPVIU-UHFFFAOYSA-N | |
Source | PubChem | |
URL | https://pubchem.ncbi.nlm.nih.gov | |
Description | Data deposited in or computed by PubChem | |
Canonical SMILES |
C1=C(C=C(N=C1Cl)Cl)Cl | |
Source | PubChem | |
URL | https://pubchem.ncbi.nlm.nih.gov | |
Description | Data deposited in or computed by PubChem | |
Molecular Formula |
C5H2Cl3N | |
Source | PubChem | |
URL | https://pubchem.ncbi.nlm.nih.gov | |
Description | Data deposited in or computed by PubChem | |
DSSTOX Substance ID |
DTXSID80166951 | |
Record name | Pyridine, 2,4,6-trichloro- | |
Source | EPA DSSTox | |
URL | https://comptox.epa.gov/dashboard/DTXSID80166951 | |
Description | DSSTox provides a high quality public chemistry resource for supporting improved predictive toxicology. | |
Molecular Weight |
182.43 g/mol | |
Source | PubChem | |
URL | https://pubchem.ncbi.nlm.nih.gov | |
Description | Data deposited in or computed by PubChem | |
CAS No. |
16063-69-7 | |
Record name | 2,4,6-Trichloropyridine | |
Source | CAS Common Chemistry | |
URL | https://commonchemistry.cas.org/detail?cas_rn=16063-69-7 | |
Description | CAS Common Chemistry is an open community resource for accessing chemical information. Nearly 500,000 chemical substances from CAS REGISTRY cover areas of community interest, including common and frequently regulated chemicals, and those relevant to high school and undergraduate chemistry classes. This chemical information, curated by our expert scientists, is provided in alignment with our mission as a division of the American Chemical Society. | |
Explanation | The data from CAS Common Chemistry is provided under a CC-BY-NC 4.0 license, unless otherwise stated. | |
Record name | Pyridine, 2,4,6-trichloro- | |
Source | ChemIDplus | |
URL | https://pubchem.ncbi.nlm.nih.gov/substance/?source=chemidplus&sourceid=0016063697 | |
Description | ChemIDplus is a free, web search system that provides access to the structure and nomenclature authority files used for the identification of chemical substances cited in National Library of Medicine (NLM) databases, including the TOXNET system. | |
Record name | Pyridine, 2,4,6-trichloro- | |
Source | EPA DSSTox | |
URL | https://comptox.epa.gov/dashboard/DTXSID80166951 | |
Description | DSSTox provides a high quality public chemistry resource for supporting improved predictive toxicology. | |
Record name | 2,4,6-Trichloropyridine | |
Source | European Chemicals Agency (ECHA) | |
URL | https://echa.europa.eu/information-on-chemicals | |
Description | The European Chemicals Agency (ECHA) is an agency of the European Union which is the driving force among regulatory authorities in implementing the EU's groundbreaking chemicals legislation for the benefit of human health and the environment as well as for innovation and competitiveness. | |
Explanation | Use of the information, documents and data from the ECHA website is subject to the terms and conditions of this Legal Notice, and subject to other binding limitations provided for under applicable law, the information, documents and data made available on the ECHA website may be reproduced, distributed and/or used, totally or in part, for non-commercial purposes provided that ECHA is acknowledged as the source: "Source: European Chemicals Agency, http://echa.europa.eu/". Such acknowledgement must be included in each copy of the material. ECHA permits and encourages organisations and individuals to create links to the ECHA website under the following cumulative conditions: Links can only be made to webpages that provide a link to the Legal Notice page. | |
Synthesis routes and methods
Procedure details
Retrosynthesis Analysis
AI-Powered Synthesis Planning: Our tool employs the Template_relevance Pistachio, Template_relevance Bkms_metabolic, Template_relevance Pistachio_ringbreaker, Template_relevance Reaxys, Template_relevance Reaxys_biocatalysis model, leveraging a vast database of chemical reactions to predict feasible synthetic routes.
One-Step Synthesis Focus: Specifically designed for one-step synthesis, it provides concise and direct routes for your target compounds, streamlining the synthesis process.
Accurate Predictions: Utilizing the extensive PISTACHIO, BKMS_METABOLIC, PISTACHIO_RINGBREAKER, REAXYS, REAXYS_BIOCATALYSIS database, our tool offers high-accuracy predictions, reflecting the latest in chemical research and data.
Strategy Settings
Precursor scoring | Relevance Heuristic |
---|---|
Min. plausibility | 0.01 |
Model | Template_relevance |
Template Set | Pistachio/Bkms_metabolic/Pistachio_ringbreaker/Reaxys/Reaxys_biocatalysis |
Top-N result to add to graph | 6 |
Feasible Synthetic Routes
Q1: Can you elaborate on the regioselectivity challenges in substituting chlorine atoms in 2,4,6-trichloropyridine?
A: Without any modification, this compound undergoes nucleophilic substitution primarily at the 4-position. [, ] This predictable but limited reactivity restricts the diversity of molecules that can be synthesized. The research highlights the challenge of accessing substitutions at the 2- and 6-positions, which are sterically less accessible and electronically less favored. []
Q2: How does the introduction of a trialkylsilyl group solve this regioselectivity problem?
A: Introducing a bulky trialkylsilyl group at the 3-position of this compound effectively blocks the 4-position, both sterically and electronically. [, ] This steric hindrance and electronic influence force the incoming nucleophile to react with the more accessible chlorine at the 6-position. Once the desired substitution at the 6-position is achieved, the trialkylsilyl group can be easily removed, leaving behind a product with the nucleophile specifically at the 6-position. [] This strategy allows chemists to access a wider range of substituted pyridine derivatives that were previously difficult to synthesize.
Disclaimer and Information on In-Vitro Research Products
Please be aware that all articles and product information presented on BenchChem are intended solely for informational purposes. The products available for purchase on BenchChem are specifically designed for in-vitro studies, which are conducted outside of living organisms. In-vitro studies, derived from the Latin term "in glass," involve experiments performed in controlled laboratory settings using cells or tissues. It is important to note that these products are not categorized as medicines or drugs, and they have not received approval from the FDA for the prevention, treatment, or cure of any medical condition, ailment, or disease. We must emphasize that any form of bodily introduction of these products into humans or animals is strictly prohibited by law. It is essential to adhere to these guidelines to ensure compliance with legal and ethical standards in research and experimentation.