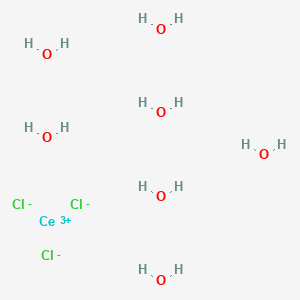
Cerous chloride heptahydrate
- Click on QUICK INQUIRY to receive a quote from our team of experts.
- With the quality product at a COMPETITIVE price, you can focus more on your research.
Overview
Description
Cerous chloride heptahydrate, also known as cerium(III) chloride heptahydrate, is a compound of cerium and chlorine with the chemical formula CeCl₃·7H₂O. It is a white, hygroscopic salt that rapidly absorbs water from the atmosphere to form a hydrate. This compound is highly soluble in water and, when anhydrous, it is soluble in ethanol and acetone .
Mechanism of Action
Target of Action
Cerous chloride heptahydrate, also known as Cerium(III) chloride heptahydrate, is a compound of cerium and chlorine It’s known to be used as a cerium source in various applications such as nanometal oxide synthesis and catalysis .
Mode of Action
It’s known to be a mild lewis acid . It participates in the allylation of aldehydes . Cerium(III) chloride heptahydrate in combination with sodium iodide supported on silica gel efficiently promotes Michael-type addition .
Biochemical Pathways
Its role in the synthesis of nanometal oxides suggests that it may influence pathways related to material synthesis and catalysis .
Pharmacokinetics
It’s known to be highly soluble in water, and when anhydrous, it is soluble in ethanol and acetone . This suggests that it could have good bioavailability, but further studies are needed to confirm this.
Result of Action
It’s known to be used as a precursor to prepare cerium oxide nanoparticles for biomedical applications and photocatalytic degradation . It can also be used as a solution to fabricate thin films of CeO2 on glass substrates by the spray pyrolysis process .
Action Environment
The action, efficacy, and stability of this compound can be influenced by environmental factors. It is a white hygroscopic salt; it rapidly absorbs water on exposure to moist air to form a hydrate . Therefore, the presence of moisture in the environment can affect its stability. Furthermore, its solubility in different solvents suggests that the solvent environment can also influence its action and efficacy .
Preparation Methods
Synthetic Routes and Reaction Conditions: Cerous chloride heptahydrate can be synthesized by reacting cerium(IV) oxide (CeO₂) with hydrochloric acid (HCl). The reaction typically involves dissolving cerium(IV) oxide in hydrochloric acid, followed by heating and stirring. The resulting solution is then filtered and evaporated to obtain this compound crystals .
Industrial Production Methods: In industrial settings, this compound is produced by the same method but on a larger scale. The process involves careful control of reaction conditions to ensure high purity and yield. The product is then dried and packaged for various applications .
Types of Reactions:
Reduction: this compound can act as a reducing agent in organic synthesis, often used in place of sodium borohydride.
Common Reagents and Conditions:
Reducing Agent: Used in organic synthesis with reagents like sodium borohydride.
Catalyst: Acts as a catalyst in the presence of sodium iodide for Michael addition reactions.
Major Products:
Allylic Alcohols: In the Luche reaction, this compound selectively converts carvone to allylic alcohol.
Cerium Oxide Nanoparticles: Used as a precursor to prepare cerium oxide nanoparticles.
Scientific Research Applications
Cerous chloride heptahydrate has a wide range of applications in scientific research:
Comparison with Similar Compounds
- Cerium(III) oxide (Ce₂O₃)
- Cerium(III) fluoride (CeF₃)
- Cerium(III) bromide (CeBr₃)
- Cerium(III) iodide (CeI₃)
- Lanthanum(III) chloride (LaCl₃)
- Praseodymium(III) chloride (PrCl₃)
Comparison: Cerous chloride heptahydrate is unique due to its high solubility in water and its ability to form stable hydrates. Compared to other cerium compounds, it is particularly useful as a precursor for cerium oxide nanoparticles, which have significant applications in biomedical and industrial fields .
Properties
CAS No. |
18618-55-8 |
---|---|
Molecular Formula |
CeCl3H2O |
Molecular Weight |
264.49 g/mol |
IUPAC Name |
cerium(3+);trichloride;hydrate |
InChI |
InChI=1S/Ce.3ClH.H2O/h;3*1H;1H2/q+3;;;;/p-3 |
InChI Key |
SMKFCFKIYPLYNY-UHFFFAOYSA-K |
SMILES |
O.O.O.O.O.O.O.[Cl-].[Cl-].[Cl-].[Ce+3] |
Canonical SMILES |
O.[Cl-].[Cl-].[Cl-].[Ce+3] |
Key on ui other cas no. |
18618-55-8 |
Pictograms |
Irritant |
Synonyms |
CeCl3 cerium trichloride cerium(III) chloride cerous chloride cerous chloride heptahydrate cerous chloride hexahydrate cerous chloride octahydrate cerous chloride, 141Ce-labeled cerous chloride, 144Ce-labeled |
Origin of Product |
United States |
Retrosynthesis Analysis
AI-Powered Synthesis Planning: Our tool employs the Template_relevance Pistachio, Template_relevance Bkms_metabolic, Template_relevance Pistachio_ringbreaker, Template_relevance Reaxys, Template_relevance Reaxys_biocatalysis model, leveraging a vast database of chemical reactions to predict feasible synthetic routes.
One-Step Synthesis Focus: Specifically designed for one-step synthesis, it provides concise and direct routes for your target compounds, streamlining the synthesis process.
Accurate Predictions: Utilizing the extensive PISTACHIO, BKMS_METABOLIC, PISTACHIO_RINGBREAKER, REAXYS, REAXYS_BIOCATALYSIS database, our tool offers high-accuracy predictions, reflecting the latest in chemical research and data.
Strategy Settings
Precursor scoring | Relevance Heuristic |
---|---|
Min. plausibility | 0.01 |
Model | Template_relevance |
Template Set | Pistachio/Bkms_metabolic/Pistachio_ringbreaker/Reaxys/Reaxys_biocatalysis |
Top-N result to add to graph | 6 |
Feasible Synthetic Routes
Q1: What is the role of cerous chloride heptahydrate in the synthesis of quinolone medicaments?
A1: this compound acts as a catalyst in the synthesis of quinolone medicaments, specifically in the piperazidine reduction of quinolone carboxylate nuclear parent with piperazidine derivatives []. This reaction is crucial for introducing the piperazine ring, a key structural feature for the antibacterial activity of quinolones.
Q2: What are the advantages of using this compound in this synthesis method compared to conventional methods?
A2: The research paper highlights several advantages of using this compound as a catalyst in this specific synthesis method []:
Disclaimer and Information on In-Vitro Research Products
Please be aware that all articles and product information presented on BenchChem are intended solely for informational purposes. The products available for purchase on BenchChem are specifically designed for in-vitro studies, which are conducted outside of living organisms. In-vitro studies, derived from the Latin term "in glass," involve experiments performed in controlled laboratory settings using cells or tissues. It is important to note that these products are not categorized as medicines or drugs, and they have not received approval from the FDA for the prevention, treatment, or cure of any medical condition, ailment, or disease. We must emphasize that any form of bodily introduction of these products into humans or animals is strictly prohibited by law. It is essential to adhere to these guidelines to ensure compliance with legal and ethical standards in research and experimentation.