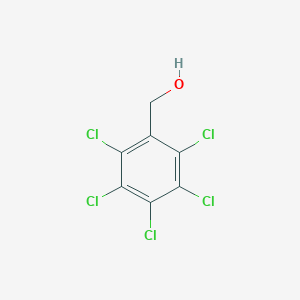
2,3,4,5,6-Pentachlorobenzenemethanol
Overview
Description
2,3,4,5,6-Pentachlorobenzenemethanol (PCBM) is a synthetic organic compound that is widely used in scientific research applications. It is known for its unique chemical properties and is used as a reagent in several laboratory experiments.
Scientific Research Applications
Reductive Dechlorination and Environmental Remediation
Research has shown that compounds like 2,3,4,5,6-pentachlorobenzenemethanol can undergo reductive dechlorination, which is significant in environmental chemistry. For example, Assaf-Anid, Nies, and Vogel (1992) demonstrated the reductive dechlorination of polychlorinated biphenyls and hexachlorobenzene using vitamin B12 in an aqueous biomimetic system (Assaf-Anid, Nies, & Vogel, 1992). This process is crucial for the detoxification of hazardous environmental pollutants.
Degradation of Toxic Compounds
The degradation of toxic compounds such as pentachlorophenol (PCP) has been explored using novel oxidants. Zamora-Garcia et al. (2017) investigated the use of electrochemically generated Ag(OH)4- as a strong oxidizing agent for treating PCP, highlighting the importance of such compounds in environmental remediation (Zamora-Garcia et al., 2017).
Catalytic Reduction and Dechlorination
Another significant application is in the catalytic reduction and dechlorination of chlorinated compounds. Lei et al. (2021) proposed a strategy for the rapid and complete dechlorination of highly-chlorinated benzenes using palladium with low content deposited g-C3N4 as a catalyst. This method is particularly effective for compounds like pentachlorobenzene (Lei et al., 2021).
Phototransformation and Environmental Impacts
The phototransformation of compounds like pentachlorophenol (PCP) has been studied for its environmental impact. Piccinini, Pichat, and Guillard (1998) investigated the phototransformation of thin layers of PCP under UV irradiation, demonstrating the formation of various chlorinated and non-chlorinated products, relevant in environmental contexts (Piccinini, Pichat, & Guillard, 1998).
properties
CAS RN |
16022-69-8 |
---|---|
Molecular Formula |
C7H3Cl5O |
Molecular Weight |
280.4 g/mol |
IUPAC Name |
(2,3,4,5,6-pentachlorophenyl)methanol |
InChI |
InChI=1S/C7H3Cl5O/c8-3-2(1-13)4(9)6(11)7(12)5(3)10/h13H,1H2 |
InChI Key |
RVCKCEDKBVEEHL-UHFFFAOYSA-N |
SMILES |
C(C1=C(C(=C(C(=C1Cl)Cl)Cl)Cl)Cl)O |
Canonical SMILES |
C(C1=C(C(=C(C(=C1Cl)Cl)Cl)Cl)Cl)O |
melting_point |
193°C |
Other CAS RN |
16022-69-8 |
physical_description |
Solid |
solubility |
7.13e-06 M 0.002 mg/mL at 20 °C |
synonyms |
(2,3,4,5,6-pentachlorophenyl)methanol |
Origin of Product |
United States |
Synthesis routes and methods I
Procedure details
Synthesis routes and methods II
Procedure details
Retrosynthesis Analysis
AI-Powered Synthesis Planning: Our tool employs the Template_relevance Pistachio, Template_relevance Bkms_metabolic, Template_relevance Pistachio_ringbreaker, Template_relevance Reaxys, Template_relevance Reaxys_biocatalysis model, leveraging a vast database of chemical reactions to predict feasible synthetic routes.
One-Step Synthesis Focus: Specifically designed for one-step synthesis, it provides concise and direct routes for your target compounds, streamlining the synthesis process.
Accurate Predictions: Utilizing the extensive PISTACHIO, BKMS_METABOLIC, PISTACHIO_RINGBREAKER, REAXYS, REAXYS_BIOCATALYSIS database, our tool offers high-accuracy predictions, reflecting the latest in chemical research and data.
Strategy Settings
Precursor scoring | Relevance Heuristic |
---|---|
Min. plausibility | 0.01 |
Model | Template_relevance |
Template Set | Pistachio/Bkms_metabolic/Pistachio_ringbreaker/Reaxys/Reaxys_biocatalysis |
Top-N result to add to graph | 6 |
Feasible Synthetic Routes
Disclaimer and Information on In-Vitro Research Products
Please be aware that all articles and product information presented on BenchChem are intended solely for informational purposes. The products available for purchase on BenchChem are specifically designed for in-vitro studies, which are conducted outside of living organisms. In-vitro studies, derived from the Latin term "in glass," involve experiments performed in controlled laboratory settings using cells or tissues. It is important to note that these products are not categorized as medicines or drugs, and they have not received approval from the FDA for the prevention, treatment, or cure of any medical condition, ailment, or disease. We must emphasize that any form of bodily introduction of these products into humans or animals is strictly prohibited by law. It is essential to adhere to these guidelines to ensure compliance with legal and ethical standards in research and experimentation.