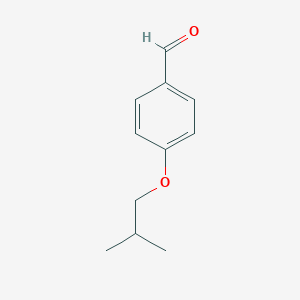
4-Isobutoxybenzaldehyde
Overview
Description
4-Isobutoxybenzaldehyde (CAS: 18962-07-7) is an aromatic aldehyde with the molecular formula C₁₁H₁₄O₂ and a molecular weight of 178.23 g/mol. It is synthesized via nucleophilic substitution of p-hydroxybenzaldehyde with 1-bromo-2-methylpropane in N,N-dimethylformamide (DMF) at 85°C, followed by oxime formation with hydroxylamine in ethanol . The compound crystallizes in a monoclinic system (space group P2₁/n) with unit cell parameters a = 13.5152(2) Å, b = 6.19120(10) Å, c = 14.3917(2) Å, and β = 115.467(2)°, forming hydrogen-bonded dimers that stabilize its three-dimensional network . Its applications span organic synthesis intermediates, pharmaceutical precursors, and materials science due to its reactive aldehyde group and bulky isobutoxy substituent.
Preparation Methods
Williamson Ether Synthesis
Reaction Overview
The Williamson ether synthesis remains the most widely employed method for introducing alkoxy groups onto aromatic systems. For 4-isobutoxybenzaldehyde, this method involves the reaction of 4-hydroxybenzaldehyde with isobutyl bromide in the presence of a strong base.
Mechanism
-
Deprotonation : The phenolic oxygen of 4-hydroxybenzaldehyde is deprotonated by a base (e.g., K₂CO₃ or NaOH), forming a phenoxide ion.
-
Nucleophilic Substitution : The phenoxide ion attacks the electrophilic carbon of isobutyl bromide, displacing the bromide ion and forming the ether linkage.
Optimization Parameters
-
Base Selection : Potassium carbonate (K₂CO₃) in acetone or DMF is preferred for its mildness and solubility.
-
Temperature : Reflux conditions (60–80°C) enhance reaction rates without promoting aldehyde degradation.
-
Molar Ratio : A 1:1.2 molar ratio of 4-hydroxybenzaldehyde to isobutyl bromide minimizes side reactions.
Yield and Scalability
Typical yields range from 65% to 85%, with scalability demonstrated in batch processes exceeding 10 kg. Purification via recrystallization (ethanol/water) or column chromatography (hexane:ethyl acetate) ensures high purity (>98%).
Ullmann Coupling
Reaction Overview
The Ullmann coupling leverages copper catalysts to facilitate the formation of aryl ethers, offering an alternative route for substrates sensitive to strongly basic conditions.
Mechanism
-
Oxidative Addition : A copper(I) catalyst (e.g., CuI) coordinates with 4-iodobenzaldehyde, forming an aryl-copper intermediate.
-
Alcohol Activation : Isobutanol is deprotonated by a base (e.g., Cs₂CO₃), generating an alkoxide ion.
-
Coupling : The aryl-copper intermediate reacts with the alkoxide, yielding this compound and regenerating the catalyst.
Optimization Parameters
-
Catalyst Loading : 10 mol% CuI ensures efficient turnover.
-
Solvent : Dimethylformamide (DMF) or dimethyl sulfoxide (DMSO) enhances solubility at 100–120°C.
-
Ligands : 1,10-Phenanthroline improves catalytic activity by stabilizing copper intermediates.
Yield and Scalability
Yields of 70–90% are achievable, though scalability is limited by the cost of iodinated precursors. Microwave-assisted protocols reduce reaction times from 24 hours to 2–4 hours.
Mitsunobu Reaction
Reaction Overview
The Mitsunobu reaction enables the coupling of alcohols with phenols under mild conditions, utilizing triphenylphosphine (PPh₃) and diethyl azodicarboxylate (DEAD).
Mechanism
-
Redox Activation : DEAD oxidizes PPh₃ to triphenylphosphine oxide, generating a protonated intermediate.
-
Alcohol Activation : Isobutanol is activated via proton transfer, forming a reactive alkoxyphosphonium ion.
-
Ether Formation : The alkoxyphosphonium ion reacts with 4-hydroxybenzaldehyde, displacing the phosphine oxide and forming the ether bond.
Optimization Parameters
-
Solvent : Tetrahydrofuran (THF) or dichloromethane (DCM) at 0–25°C prevents aldehyde oxidation.
-
Stoichiometry : Equimolar amounts of DEAD and PPh₃ are critical for complete conversion.
Yield and Scalability
Yields exceed 90% in laboratory settings, but industrial adoption is hindered by the high cost of DEAD and phosphine byproducts.
Nucleophilic Aromatic Substitution
Reaction Overview
This method exploits electron-deficient aryl halides, where a nitro group at the para position activates the ring for substitution by isobutoxide ions.
Mechanism
-
Activation : The nitro group withdraws electron density, rendering the aryl halide susceptible to nucleophilic attack.
-
Substitution : Isobutoxide ions displace the halide (e.g., Cl or F), forming the ether linkage.
Optimization Parameters
-
Leaving Group : Fluorine (4-fluorobenzaldehyde) offers superior reactivity over chlorine.
-
Temperature : Reactions proceed at 120–150°C in polar aprotic solvents (e.g., NMP).
Yield and Scalability
Yields of 50–70% are typical, with scalability limited by the need for high temperatures and specialized equipment.
Phase Transfer Catalysis (PTC)
Reaction Overview
PTC facilitates reactions between aqueous bases and organic substrates, enabling efficient alkylation of 4-hydroxybenzaldehyde in biphasic systems.
Mechanism
-
Base Activation : Aqueous NaOH deprotonates 4-hydroxybenzaldehyde, forming a phenoxide ion.
-
Catalytic Transfer : A phase transfer catalyst (e.g., tetrabutylammonium bromide) shuttles the phenoxide into the organic phase.
-
Alkylation : The phenoxide reacts with isobutyl bromide, forming the ether product.
Optimization Parameters
-
Catalyst Loading : 5 mol% tetrabutylammonium bromide maximizes interfacial transfer.
-
Solvent System : Toluene/water mixtures (1:1 v/v) optimize phase separation.
Yield and Scalability
Yields of 75–85% are achievable, with industrial scalability demonstrated in continuous-flow reactors.
Comparative Analysis of Preparation Methods
Method | Yield (%) | Temperature (°C) | Catalyst/Base | Scalability |
---|---|---|---|---|
Williamson Synthesis | 65–85 | 60–80 | K₂CO₃ | High |
Ullmann Coupling | 70–90 | 100–120 | CuI, Cs₂CO₃ | Moderate |
Mitsunobu Reaction | >90 | 0–25 | DEAD, PPh₃ | Low |
Nucleophilic Substitution | 50–70 | 120–150 | None | Low |
Phase Transfer Catalysis | 75–85 | 25–40 | Tetrabutylammonium bromide | High |
Scientific Research Applications
Pharmaceutical Applications
Drug Intermediates
4-Isobutoxybenzaldehyde serves as an important intermediate in the synthesis of various pharmaceutical compounds. One notable derivative is this compound oxime, which has been identified as a crucial precursor in drug development . The oxime derivative exhibits potential therapeutic properties and has been studied for its efficacy against certain types of cancer.
Case Study: Aldehyde Dehydrogenase Inhibitors
Research indicates that aldehyde dehydrogenases (ALDHs) are overexpressed in several tumor types, making them targets for cancer therapy. Compounds similar to this compound have been explored for their ability to inhibit ALDH isoforms, which could lead to enhanced cytotoxicity against cancer cells. For instance, analogues derived from benzaldehyde scaffolds have shown promising results in inhibiting ALDH activity in prostate cancer cell lines .
Agrochemical Applications
Pesticides and Herbicides
The compound is also utilized in the formulation of agrochemicals. Its derivatives can enhance the efficacy of pesticides and herbicides by improving their stability and solubility in agricultural formulations. This application underscores the importance of this compound in sustainable agriculture practices.
Organic Synthesis
Building Block for Organic Compounds
In organic chemistry, this compound acts as a versatile building block for synthesizing various organic compounds. Its functional groups allow for further chemical modifications, making it valuable in creating more complex molecules used in different industrial applications.
Mechanism of Action
The mechanism of action of 4-Isobutoxybenzaldehyde involves its interaction with specific molecular targets. For instance, it may act as an inhibitor of certain enzymes or receptors, modulating biochemical pathways. The exact molecular targets and pathways can vary depending on the specific application and context of use .
Comparison with Similar Compounds
Comparison with Structurally Similar Compounds
The following table and analysis compare 4-isobutoxybenzaldehyde with analogous aldehydes in terms of structure, physicochemical properties, and biological/industrial relevance:
Key Comparisons :
Substituent Effects on Reactivity: The isobutoxy group in this compound introduces steric hindrance, reducing nucleophilic attack rates compared to smaller substituents like methoxy (-OCH₃) or hydroxyl (-OH) .
Physicochemical Properties :
- 4-Hydroxybenzaldehyde has higher polarity due to its hydroxyl group, leading to greater water solubility (1.3 g/L at 25°C) compared to the hydrophobic isobutoxy derivative .
- The benzodioxane ring in 2,3-dihydro-1,4-benzodioxine-5-carbaldehyde enhances thermal stability (boiling point: 283°C) compared to this compound .
Biological Activity: 4-Hydroxybenzaldehyde demonstrates significant bioactivity, including inhibition of pro-inflammatory cytokines (e.g., TNF-α) and antimicrobial effects against Staphylococcus aureus .
Safety and Handling :
- 4-(Bromomethyl)benzaldehyde poses higher risks due to its bromomethyl group, requiring stringent safety measures (e.g., eye flushing for 15 minutes upon contact) .
- This compound and 4-methoxybenzaldehyde are less hazardous but still necessitate standard aldehyde precautions (e.g., ventilation, gloves) .
Biological Activity
4-Isobutoxybenzaldehyde, a compound with the chemical formula CHO, is an aromatic aldehyde that has garnered attention for its potential biological activities. This article explores the synthesis, biological properties, and relevant research findings associated with this compound.
This compound is characterized by an isobutoxy group (-O-C(CH)H) attached to a benzaldehyde structure. This unique structure may influence its biological activity, particularly in terms of its interactions with various biological systems.
Cytotoxicity Studies
A study evaluating the cytotoxic effects of synthetic cathinones, which share structural similarities with this compound, found that certain derivatives exhibited significant cytotoxicity in human cell lines. Although direct studies on this compound are scarce, the implications of its structural characteristics suggest potential cytotoxic effects that warrant further investigation. The IC values for related compounds ranged from 18 to 65 µM in various human cell lines, indicating a need to explore the cytotoxic profile of this compound in similar assays .
Synthesis and Evaluation
Research has focused on synthesizing and evaluating the biological activities of various benzaldehyde derivatives. For example, a study synthesized several aryl-alkyl derivatives and tested their antimicrobial efficacy. While specific data on this compound was not highlighted, the general findings suggest that modifications to the benzaldehyde structure can lead to enhanced biological activities .
The mechanism by which benzaldehyde derivatives exert their biological effects often involves interactions with cellular receptors or enzymes. For instance, studies have shown that certain derivatives can act as inhibitors of key enzymes involved in microbial metabolism or cell proliferation. Exploring the specific interactions of this compound with biological targets could provide insights into its potential therapeutic applications.
Data Table: Biological Activity Comparison
Q & A
Basic Research Questions
Q. How can researchers optimize the synthesis of 4-Isobutoxybenzaldehyde to improve yield and purity?
Methodological Answer: Optimization involves selecting appropriate precursors (e.g., 4-hydroxybenzaldehyde derivatives) and reaction conditions. For example, alkylation of 4-hydroxybenzaldehyde with isobutyl bromide under basic conditions (e.g., K₂CO₃ in DMF) can yield this compound. Reaction temperature (80–100°C), solvent polarity, and catalyst choice (e.g., phase-transfer catalysts) significantly affect yield . Purification via column chromatography or recrystallization (using ethanol/water mixtures) enhances purity. Validate synthetic routes using databases like Reaxys or SciFinder to compare with analogous benzaldehyde derivatives .
Q. What spectroscopic techniques are most effective for characterizing this compound?
Methodological Answer:
- NMR Spectroscopy: ¹H NMR confirms the isobutoxy group (δ 1.0–1.2 ppm for -CH(CH₃)₂ protons) and aldehyde proton (δ ~9.8 ppm). ¹³C NMR identifies carbonyl (δ ~190 ppm) and aromatic carbons .
- IR Spectroscopy: Stretching vibrations for C=O (~1680 cm⁻¹) and aryl ether (C-O-C, ~1250 cm⁻¹) confirm functional groups .
- Mass Spectrometry: High-resolution MS (HRMS) verifies molecular formula (C₁₁H₁₄O₂) and fragmentation patterns .
Q. How should solubility data for this compound be experimentally determined and validated?
Methodological Answer: Use gravimetric or UV-Vis methods in solvents like water, ethanol, and DMSO. For low solubility, apply the shake-flask method: saturate the solvent with the compound, filter, and quantify via HPLC or gravimetry. Compare results with analogous compounds (e.g., 4-methoxybenzaldehyde solubility: 8.45 mg/mL in water at 25°C) . Address discrepancies by standardizing temperature, agitation, and solvent purity .
Advanced Research Questions
Q. What computational methods can predict the reactivity of this compound in novel reactions?
Methodological Answer:
- Retrosynthesis Tools: AI-driven platforms (e.g., Reaxys or Pistachio) analyze feasible pathways using databases of analogous reactions, such as alkylation or oxidation of benzaldehyde derivatives .
- DFT Calculations: Predict electronic properties (e.g., HOMO/LUMO energies) to assess nucleophilic/electrophilic behavior. Compare with experimental kinetic data (e.g., reaction rates in SN2 alkylation) .
- Molecular Dynamics (MD): Simulate solvent effects on reaction mechanisms, such as solvolysis in polar aprotic solvents .
Q. How can researchers resolve contradictions in reported catalytic efficiencies for this compound reactions?
Methodological Answer:
- Systematic Replication: Reproduce experiments under identical conditions (solvent, catalyst loading, temperature). For example, discrepancies in palladium-catalyzed coupling may arise from trace moisture or oxygen .
- Error Analysis: Quantify uncertainties in instrumentation (e.g., GC-MS calibration drift) or procedural variability (e.g., stirring efficiency) .
- Meta-Analysis: Aggregate data from multiple studies (e.g., CAS Common Chemistry) and apply statistical models (e.g., ANOVA) to identify outliers .
Q. What strategies validate the environmental safety of this compound in biomedical applications?
Methodological Answer:
- Toxicology Profiling: Use read-across models (grouping with structurally similar compounds like 4-hydroxybenzaldehyde) to infer toxicity. Assess Ames test data for mutagenicity and acute toxicity in vitro (e.g., HepG2 cell viability assays) .
- Metabolic Stability Studies: Incubate with liver microsomes to identify metabolites (e.g., oxidation of the aldehyde group) via LC-HRMS .
- Environmental Fate Modeling: Predict biodegradation pathways using EPI Suite or OECD QSAR Toolbox .
Q. Data Presentation and Analysis Guidelines
- Raw Data Handling: Store large datasets (e.g., reaction yields, spectroscopic peaks) in appendices. Highlight processed data (e.g., normalized chromatograms) in the main text .
- Uncertainty Reporting: Include error bars for replicate experiments and discuss limitations (e.g., instrument detection limits for trace impurities) .
Properties
IUPAC Name |
4-(2-methylpropoxy)benzaldehyde | |
---|---|---|
Source | PubChem | |
URL | https://pubchem.ncbi.nlm.nih.gov | |
Description | Data deposited in or computed by PubChem | |
InChI |
InChI=1S/C11H14O2/c1-9(2)8-13-11-5-3-10(7-12)4-6-11/h3-7,9H,8H2,1-2H3 | |
Source | PubChem | |
URL | https://pubchem.ncbi.nlm.nih.gov | |
Description | Data deposited in or computed by PubChem | |
InChI Key |
PWASYRSZCSTUIW-UHFFFAOYSA-N | |
Source | PubChem | |
URL | https://pubchem.ncbi.nlm.nih.gov | |
Description | Data deposited in or computed by PubChem | |
Canonical SMILES |
CC(C)COC1=CC=C(C=C1)C=O | |
Source | PubChem | |
URL | https://pubchem.ncbi.nlm.nih.gov | |
Description | Data deposited in or computed by PubChem | |
Molecular Formula |
C11H14O2 | |
Source | PubChem | |
URL | https://pubchem.ncbi.nlm.nih.gov | |
Description | Data deposited in or computed by PubChem | |
DSSTOX Substance ID |
DTXSID70290416 | |
Record name | 4-isobutoxybenzaldehyde | |
Source | EPA DSSTox | |
URL | https://comptox.epa.gov/dashboard/DTXSID70290416 | |
Description | DSSTox provides a high quality public chemistry resource for supporting improved predictive toxicology. | |
Molecular Weight |
178.23 g/mol | |
Source | PubChem | |
URL | https://pubchem.ncbi.nlm.nih.gov | |
Description | Data deposited in or computed by PubChem | |
CAS No. |
18962-07-7 | |
Record name | 4-(2-Methylpropoxy)benzaldehyde | |
Source | CAS Common Chemistry | |
URL | https://commonchemistry.cas.org/detail?cas_rn=18962-07-7 | |
Description | CAS Common Chemistry is an open community resource for accessing chemical information. Nearly 500,000 chemical substances from CAS REGISTRY cover areas of community interest, including common and frequently regulated chemicals, and those relevant to high school and undergraduate chemistry classes. This chemical information, curated by our expert scientists, is provided in alignment with our mission as a division of the American Chemical Society. | |
Explanation | The data from CAS Common Chemistry is provided under a CC-BY-NC 4.0 license, unless otherwise stated. | |
Record name | NSC 68517 | |
Source | ChemIDplus | |
URL | https://pubchem.ncbi.nlm.nih.gov/substance/?source=chemidplus&sourceid=0018962077 | |
Description | ChemIDplus is a free, web search system that provides access to the structure and nomenclature authority files used for the identification of chemical substances cited in National Library of Medicine (NLM) databases, including the TOXNET system. | |
Record name | 18962-07-7 | |
Source | DTP/NCI | |
URL | https://dtp.cancer.gov/dtpstandard/servlet/dwindex?searchtype=NSC&outputformat=html&searchlist=68517 | |
Description | The NCI Development Therapeutics Program (DTP) provides services and resources to the academic and private-sector research communities worldwide to facilitate the discovery and development of new cancer therapeutic agents. | |
Explanation | Unless otherwise indicated, all text within NCI products is free of copyright and may be reused without our permission. Credit the National Cancer Institute as the source. | |
Record name | 4-isobutoxybenzaldehyde | |
Source | EPA DSSTox | |
URL | https://comptox.epa.gov/dashboard/DTXSID70290416 | |
Description | DSSTox provides a high quality public chemistry resource for supporting improved predictive toxicology. | |
Record name | 18962-07-7 | |
Source | European Chemicals Agency (ECHA) | |
URL | https://echa.europa.eu/information-on-chemicals | |
Description | The European Chemicals Agency (ECHA) is an agency of the European Union which is the driving force among regulatory authorities in implementing the EU's groundbreaking chemicals legislation for the benefit of human health and the environment as well as for innovation and competitiveness. | |
Explanation | Use of the information, documents and data from the ECHA website is subject to the terms and conditions of this Legal Notice, and subject to other binding limitations provided for under applicable law, the information, documents and data made available on the ECHA website may be reproduced, distributed and/or used, totally or in part, for non-commercial purposes provided that ECHA is acknowledged as the source: "Source: European Chemicals Agency, http://echa.europa.eu/". Such acknowledgement must be included in each copy of the material. ECHA permits and encourages organisations and individuals to create links to the ECHA website under the following cumulative conditions: Links can only be made to webpages that provide a link to the Legal Notice page. | |
Synthesis routes and methods I
Procedure details
Synthesis routes and methods II
Procedure details
Retrosynthesis Analysis
AI-Powered Synthesis Planning: Our tool employs the Template_relevance Pistachio, Template_relevance Bkms_metabolic, Template_relevance Pistachio_ringbreaker, Template_relevance Reaxys, Template_relevance Reaxys_biocatalysis model, leveraging a vast database of chemical reactions to predict feasible synthetic routes.
One-Step Synthesis Focus: Specifically designed for one-step synthesis, it provides concise and direct routes for your target compounds, streamlining the synthesis process.
Accurate Predictions: Utilizing the extensive PISTACHIO, BKMS_METABOLIC, PISTACHIO_RINGBREAKER, REAXYS, REAXYS_BIOCATALYSIS database, our tool offers high-accuracy predictions, reflecting the latest in chemical research and data.
Strategy Settings
Precursor scoring | Relevance Heuristic |
---|---|
Min. plausibility | 0.01 |
Model | Template_relevance |
Template Set | Pistachio/Bkms_metabolic/Pistachio_ringbreaker/Reaxys/Reaxys_biocatalysis |
Top-N result to add to graph | 6 |
Feasible Synthetic Routes
Disclaimer and Information on In-Vitro Research Products
Please be aware that all articles and product information presented on BenchChem are intended solely for informational purposes. The products available for purchase on BenchChem are specifically designed for in-vitro studies, which are conducted outside of living organisms. In-vitro studies, derived from the Latin term "in glass," involve experiments performed in controlled laboratory settings using cells or tissues. It is important to note that these products are not categorized as medicines or drugs, and they have not received approval from the FDA for the prevention, treatment, or cure of any medical condition, ailment, or disease. We must emphasize that any form of bodily introduction of these products into humans or animals is strictly prohibited by law. It is essential to adhere to these guidelines to ensure compliance with legal and ethical standards in research and experimentation.