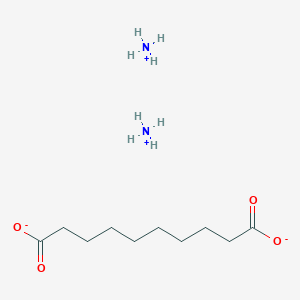
Ammonium sebacate
Overview
Description
Ammonium sebacate (CAS 19402-63-2) is the ammonium salt of sebacic acid, a dicarboxylic acid with the formula $(\text{NH}4)2\text{C}{10}\text{H}{16}\text{O}_4$. It is synthesized by neutralizing sebacic acid with ammonium hydroxide, often utilized in precipitation processes for rare earth element separations . Key applications include its role as an electrolyte in aluminum electrolytic capacitors, where it demonstrates moderate conductivity ($1.7 \times 10^{-3} \, \text{S/cm}$ at 30°C) and stability up to 469 V . Its pH (5.9) and thermal decomposition profile make it suitable for industrial formulations, though alternatives with enhanced performance are increasingly sought .
Preparation Methods
Synthetic Routes and Reaction Conditions: Ammonium sebacate can be synthesized through the esterification reaction of this compound and ethylene glycol . The reaction conditions involve controlling the temperature and heat preservation time to enhance the solubility of this compound in ethylene glycol . This method ensures high conductivity and stability of the resulting product .
Industrial Production Methods: In industrial settings, this compound is produced by reacting decanedioic acid with ammonium hydroxide. The reaction is typically carried out in an aqueous medium, followed by crystallization to obtain the pure compound . The process is straightforward, cost-effective, and suitable for large-scale production .
Chemical Reactions Analysis
Types of Reactions: Ammonium sebacate undergoes various chemical reactions, including:
Oxidation: It can be oxidized to form sebacic acid and other by-products.
Reduction: Under specific conditions, it can be reduced to form different amine derivatives.
Substitution: It can participate in substitution reactions, particularly with halogens and other reactive groups.
Common Reagents and Conditions:
Oxidation: Common oxidizing agents include potassium permanganate and hydrogen peroxide.
Reduction: Reducing agents such as lithium aluminum hydride are used.
Substitution: Halogenating agents like chlorine or bromine are commonly employed.
Major Products Formed:
Oxidation: Sebacic acid and water.
Reduction: Amine derivatives and water.
Substitution: Halogenated sebacate compounds.
Scientific Research Applications
Biological Applications
Histone Deacetylase Inhibition
One of the most significant areas of research involves ammonium sebacate's ability to inhibit histone deacetylases (HDACs). HDACs play a crucial role in regulating gene expression by removing acetyl groups from histones. Studies have shown that this compound can increase histone acetylation, potentially leading to altered gene expression patterns associated with various diseases, including cancer.
Autophagy Induction
this compound has also been investigated for its potential to induce autophagy, a cellular process that degrades and recycles damaged components. This property may be beneficial in treating neurodegenerative disorders and other diseases linked to impaired autophagy.
Material Science Applications
Corrosion Inhibition
In industrial settings, this compound serves as an effective corrosion inhibitor in metalworking fluids. Its ability to form protective layers on metal surfaces helps prevent oxidation and deterioration, making it suitable for applications where safety and non-toxicity are paramount .
Electrolyte in Capacitors
Recent studies have explored the use of this compound as an electrolyte in aluminum electrolytic capacitors. Research indicates that incorporating this compound can enhance the dielectric performance of anodic alumina films used in these capacitors .
Environmental Applications
Water Quality Monitoring
Ammonium ions are critical indicators of water quality; thus, this compound's role as a reagent in analytical chemistry is noteworthy. It has been utilized in developing ion-selective electrodes (ISEs) for monitoring ammonium concentrations in natural waters, contributing to environmental assessments and pollution control .
Case Study 1: Corrosion Inhibition
In a study examining various corrosion inhibitors for metalworking fluids, this compound demonstrated significant effectiveness in reducing oxidation rates compared to traditional inhibitors. The results highlighted its potential for safer industrial applications due to its low toxicity profile .
Case Study 2: Autophagy Research
Research involving cellular models treated with this compound showed enhanced autophagic activity, which was linked to improved cellular health markers. This finding opens avenues for further investigation into its therapeutic potential against neurodegenerative diseases.
Mechanism of Action
The mechanism of action of ammonium sebacate involves its interaction with various molecular targets. In the context of aluminum anodization, it facilitates the formation of porous anodic films by promoting oxygen evolution . The incorporation of phosphate ions in the anodic films acts as the primary source of avalanche electrons, leading to the generation of oxygen bubbles and the formation of pores .
Comparison with Similar Compounds
Structural Comparison with Metal Sebacate Salts
Sodium Sebacate
Sodium sebacate ($\text{Na}2\text{C}{10}\text{H}{16}\text{O}4$) exhibits a distinct crystal structure compared to ammonium sebacate. X-ray diffraction reveals a shorter unit cell edge and basal reflections at higher angles, indicating a more compact lattice. Unlike this compound, sodium sebacate forms dendritic crystals under slow crystallization, complicating structural resolution .
Calcium Sebacate
Calcium sebacate ($\text{CaC}{10}\text{H}{16}\text{O}_4$) features a layered structure with intense basal peaks. Its unit cell dimensions are larger than sodium sebacate, contributing to higher thermal stability. This compound is employed in rare earth separations, where it precipitates thorium at pH 2.5, contrasting with this compound’s precipitation of rare earths at pH 9 .
Table 1: Structural Properties of Sebacate Salts
Functional Comparison with Polymeric Sebacate Derivatives
Side-Chain Polycarbon Dibasic Acid Ammonium (SCPDAA)
SCPDAA, a polymeric derivative, outperforms this compound in capacitors. It exhibits higher conductivity ($1.9 \times 10^{-3} \, \text{S/cm}$), voltage stability (483 V), and pH neutrality (6.0). After 1,500 hours at 135°C, capacitors with SCPDAA show minimal capacitance loss ($-4.61\%$) compared to this compound ($-7.71\%$) .
Bis-TMP Sebacate Derivatives
Bis-(2,2,6,6-tetramethyl-4-piperidinyl) sebacate (BTMPS) is a non-competitive antagonist at neuronal nicotinic receptors, highlighting pharmacological applications absent in this compound .
Comparative Analysis with Sebacate Esters
Dibutyl Sebacate
A plasticizer in polymers and cosmetics, dibutyl sebacate ($\text{C}{18}\text{H}{34}\text{O}4$) reduces tensile strength in transdermal films similarly to dibutyl phthalate but enables faster drug release . Its log $K{ow}$ (10.08) contrasts sharply with disodium sebacate ($-4.9$), reflecting divergent hydrophobicity .
Dioctyl Sebacate
Used in sensors and propellants, dioctyl sebacate ($\text{C}{26}\text{H}{50}\text{O}_4$) acts as a plasticizer in ammonium-selective electrodes, enhancing membrane flexibility .
Table 2: Key Esters vs. This compound
Property | This compound | Dibutyl Sebacate | Dioctyl Sebacate |
---|---|---|---|
Log $K_{ow}$ | Not reported | 10.08 | Not reported |
Application | Capacitors | Plasticizer | Sensors/Propellants |
Conductivity (S/cm) | $1.7 \times 10^{-3}$ | N/A | N/A |
Thermal Stability (°C) | ~200 | >200 | >200 |
Regulatory Challenges | Moderate | High | Moderate |
References |
Biological Activity
Ammonium sebacate, a compound formed from the neutralization of sebacic acid with ammonium hydroxide, has garnered attention for its diverse biological activities. This article explores its potential antimicrobial properties, effects on gene regulation through histone deacetylase inhibition, and its role in autophagy induction.
- Chemical Formula : C10H24N2O4
- Molecular Weight : 236.31 g/mol
- Physical Form : White crystalline solid
- Solubility : Soluble in water; pH of a 5% solution ranges from 6.5 to 7.5, indicating neutrality.
Antimicrobial Properties
Research indicates that this compound exhibits antimicrobial activity against specific bacterial and fungal strains. It has been tested against:
Microorganism | Type | Activity Observed |
---|---|---|
Escherichia coli | Bacteria | Inhibition |
Candida albicans | Fungus | Inhibition |
These findings suggest potential applications in pharmaceutical formulations and as a preservative in various products. The exact mechanisms underlying this antimicrobial activity require further investigation.
Histone Deacetylase Inhibition
One of the most significant areas of research regarding this compound is its ability to inhibit histone deacetylases (HDACs) . HDACs are enzymes that remove acetyl groups from histone proteins, influencing gene expression. Studies have shown that treatment with this compound can lead to:
- Increased histone acetylation
- Altered gene expression patterns
This modulation of HDAC activity positions this compound as a valuable tool in research focused on gene regulation and its implications in diseases such as cancer.
Induction of Autophagy
This compound has also been implicated in the induction of autophagy , a cellular process that degrades and recycles damaged components. Research findings indicate that:
- Treatment with this compound activates autophagy pathways.
- This activation may promote cellular health and survival, particularly in the context of neurodegenerative diseases and cancer.
The specific pathways through which this compound induces autophagy remain to be fully elucidated, but preliminary studies have shown promising results.
Case Studies
- Antimicrobial Activity Study : A study conducted on various microbial strains demonstrated that this compound effectively inhibited the growth of E. coli and C. albicans, suggesting its potential as an antimicrobial agent in clinical settings.
- Histone Acetylation Research : In vitro experiments revealed that cells treated with this compound exhibited significantly higher levels of acetylated histones compared to untreated controls, indicating its role as an HDAC inhibitor.
- Autophagy Induction Study : A model using neuroblastoma cells showed that treatment with this compound resulted in increased autophagic markers, suggesting enhanced cellular recycling processes.
Q & A
Q. Basic: What are the recommended synthesis and purification methodologies for ammonium sebacate in laboratory settings?
This compound is synthesized via neutralization of sebacic acid with ammonium hydroxide. A typical procedure involves:
- Dissolving sebacic acid in a polar solvent (e.g., ethanol) under controlled temperature (60–70°C).
- Slowly adding ammonium hydroxide (NH₄OH) to achieve pH 7–8, followed by solvent evaporation under reduced pressure.
- Recrystallization from ethanol-water mixtures to enhance purity.
Characterization via FTIR (C=O stretch at ~1700 cm⁻¹, NH₄⁺ bands at ~3200 cm⁻¹) and NMR (δ 1.2–1.6 ppm for methylene groups, δ 6.5–7.5 ppm for ammonium protons) confirms structural integrity .
Q. Basic: Which analytical techniques are critical for characterizing this compound’s physicochemical properties?
Key techniques include:
- Thermogravimetric Analysis (TGA) : Assess thermal stability (decomposition onset ~200°C for sebacate salts).
- Differential Scanning Calorimetry (DSC) : Identify phase transitions (e.g., melting points, glass transitions).
- Ion Chromatography : Quantify ammonium ion content.
- Dynamic Light Scattering (DLS) : Measure particle size distributions in colloidal suspensions.
Cross-referencing with analogs like poly(glycerol sebacate) ensures methodological validity .
Q. Advanced: How can researchers resolve contradictions in aerosol calibration data involving this compound analogs?
Discrepancies in particle counting efficiency (e.g., between ammonium sulfate and dioctyl sebacate aerosols) arise from differences in hydrophobicity and volatility. To address this:
- Standardize aerosol generation using atomizers or flow tube reactors.
- Validate calibration curves across multiple compositions (e.g., limonene ozonolysis products for organic aerosols).
- Apply statistical tools (ANOVA) to confirm no significant variation in counting efficiency for particles >20 nm .
Q. Advanced: What strategies are effective for using analog data to fill gaps in this compound’s toxicity profile?
For endpoints lacking experimental data (e.g., developmental toxicity):
- Select structurally similar analogs (e.g., dibutyl sebacate, diisopropyl sebacate) with validated toxicity data.
- Use QSAR models to predict endpoints like log Kow (octanol-water partition coefficient) and biodegradability.
- Apply weight-of-evidence approaches to consolidate analog data and computational predictions, ensuring regulatory acceptance .
Q. Advanced: What methodologies are robust for assessing this compound’s environmental persistence?
- Biodegradation Assays : Use OECD 301B guidelines with activated sludge inoculum. Monitor CO₂ evolution via respirometry.
- QSAR Toolbox : Predict half-life in soil/water based on ester hydrolysis rates.
- Microbial Growth Studies : Test degradation using pure cultures (e.g., Pseudomonas spp.) with sebacate as the sole carbon source. Slow biodegradation of branched sebacates (e.g., bis(2-ethylhexyl) sebacate) suggests this compound may follow similar pathways .
Q. Advanced: How can computational models enhance the study of this compound’s metabolic fate?
- ADME Prediction : Use tools like ADMET Predictor™ to estimate absorption (Caco-2 permeability) and metabolism (cytochrome P450 interactions).
- Molecular Dynamics Simulations : Model interactions with biological membranes to predict distribution.
- Metabolite Identification : Combine in silico fragmentation (e.g., MetFrag) with LC-MS/MS data to map hydrolysis products (e.g., sebacic acid, ammonium ions) .
Q. Advanced: What experimental designs optimize this compound’s application in tissue engineering scaffolds?
- Electrospinning : Blend this compound derivatives (e.g., poly(glycerol sebacate)) with PLA/PCL at ratios (e.g., 70:30) to enhance elasticity.
- In Vivo Degradation : Implant scaffolds in rodent models and monitor mass loss via micro-CT.
- Mechanical Testing : Use tensile testing (ISO 37 standards) to validate Young’s modulus (~0.1–1 MPa) and strain-to-failure (>200%) for soft tissue compatibility .
Properties
IUPAC Name |
diazanium;decanedioate | |
---|---|---|
Source | PubChem | |
URL | https://pubchem.ncbi.nlm.nih.gov | |
Description | Data deposited in or computed by PubChem | |
InChI |
InChI=1S/C10H18O4.2H3N/c11-9(12)7-5-3-1-2-4-6-8-10(13)14;;/h1-8H2,(H,11,12)(H,13,14);2*1H3 | |
Source | PubChem | |
URL | https://pubchem.ncbi.nlm.nih.gov | |
Description | Data deposited in or computed by PubChem | |
InChI Key |
SATJMZAWJRWBRX-UHFFFAOYSA-N | |
Source | PubChem | |
URL | https://pubchem.ncbi.nlm.nih.gov | |
Description | Data deposited in or computed by PubChem | |
Canonical SMILES |
C(CCCCC(=O)[O-])CCCC(=O)[O-].[NH4+].[NH4+] | |
Source | PubChem | |
URL | https://pubchem.ncbi.nlm.nih.gov | |
Description | Data deposited in or computed by PubChem | |
Molecular Formula |
C10H24N2O4 | |
Source | PubChem | |
URL | https://pubchem.ncbi.nlm.nih.gov | |
Description | Data deposited in or computed by PubChem | |
DSSTOX Substance ID |
DTXSID00648441 | |
Record name | Bisammonium decanedioate | |
Source | EPA DSSTox | |
URL | https://comptox.epa.gov/dashboard/DTXSID00648441 | |
Description | DSSTox provides a high quality public chemistry resource for supporting improved predictive toxicology. | |
Molecular Weight |
236.31 g/mol | |
Source | PubChem | |
URL | https://pubchem.ncbi.nlm.nih.gov | |
Description | Data deposited in or computed by PubChem | |
CAS No. |
19402-63-2 | |
Record name | Bisammonium decanedioate | |
Source | EPA DSSTox | |
URL | https://comptox.epa.gov/dashboard/DTXSID00648441 | |
Description | DSSTox provides a high quality public chemistry resource for supporting improved predictive toxicology. | |
Retrosynthesis Analysis
AI-Powered Synthesis Planning: Our tool employs the Template_relevance Pistachio, Template_relevance Bkms_metabolic, Template_relevance Pistachio_ringbreaker, Template_relevance Reaxys, Template_relevance Reaxys_biocatalysis model, leveraging a vast database of chemical reactions to predict feasible synthetic routes.
One-Step Synthesis Focus: Specifically designed for one-step synthesis, it provides concise and direct routes for your target compounds, streamlining the synthesis process.
Accurate Predictions: Utilizing the extensive PISTACHIO, BKMS_METABOLIC, PISTACHIO_RINGBREAKER, REAXYS, REAXYS_BIOCATALYSIS database, our tool offers high-accuracy predictions, reflecting the latest in chemical research and data.
Strategy Settings
Precursor scoring | Relevance Heuristic |
---|---|
Min. plausibility | 0.01 |
Model | Template_relevance |
Template Set | Pistachio/Bkms_metabolic/Pistachio_ringbreaker/Reaxys/Reaxys_biocatalysis |
Top-N result to add to graph | 6 |
Feasible Synthetic Routes
Disclaimer and Information on In-Vitro Research Products
Please be aware that all articles and product information presented on BenchChem are intended solely for informational purposes. The products available for purchase on BenchChem are specifically designed for in-vitro studies, which are conducted outside of living organisms. In-vitro studies, derived from the Latin term "in glass," involve experiments performed in controlled laboratory settings using cells or tissues. It is important to note that these products are not categorized as medicines or drugs, and they have not received approval from the FDA for the prevention, treatment, or cure of any medical condition, ailment, or disease. We must emphasize that any form of bodily introduction of these products into humans or animals is strictly prohibited by law. It is essential to adhere to these guidelines to ensure compliance with legal and ethical standards in research and experimentation.