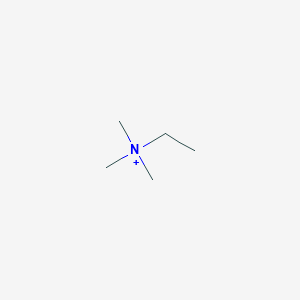
Ethyltrimethylammonium
- Click on QUICK INQUIRY to receive a quote from our team of experts.
- With the quality product at a COMPETITIVE price, you can focus more on your research.
Overview
Description
Ethyltrimethylammonium is a quarternary ammonium cation having one ethyl and three methyl substituents around the central nitrogen.
Scientific Research Applications
Biomedical Applications
1.1 Encapsulation for Diabetes Treatment
Ethyltrimethylammonium derivatives are pivotal in the development of microcapsules for encapsulating pancreatic islets, which are crucial for treating insulin deficiency in diabetes. A study demonstrated that microcapsules made from alginate and poly[2-(methacryloyloxy)ethyl]trimethylammonium chloride (PMETAC) significantly improved the viability and functionality of encapsulated islets post-transplantation. The microcapsules maintained insulin secretion for up to 90 days, compared to just 7 days for non-encapsulated islets, showcasing their potential for long-term therapeutic applications .
1.2 Antimicrobial Properties
This compound compounds exhibit notable antimicrobial activities. Research indicates that METAC demonstrates significant antifungal activity against various strains of Candida spp. and antibacterial effects against Gram-positive and Gram-negative bacteria. Minimum inhibitory concentrations (MICs) were recorded, showing effectiveness against pathogens such as Staphylococcus aureus and Escherichia coli. This makes it a promising candidate for use in medical adhesives and coatings that require antimicrobial properties .
Environmental Applications
2.1 Water Treatment
Hydrogels based on this compound derivatives have been synthesized for environmental remediation purposes, particularly in the removal of contaminants from water. These hydrogels can effectively adsorb dyes and heavy metals, contributing to cleaner water sources. For instance, bio-based hydrogels incorporating this compound have shown efficacy in adsorbing contaminant dyes like methyl orange, indicating their potential utility in wastewater treatment processes .
Material Science Applications
3.1 Development of Smart Materials
The incorporation of this compound into polymer matrices has led to the creation of smart materials with enhanced mechanical and biological properties. For example, experimental adhesives formulated with METAC have demonstrated improved antibacterial activity while maintaining structural integrity. These materials can be utilized in dental applications where infection control is critical .
3.2 Surface Modification
This compound derivatives are also employed in surface modification techniques to improve biocompatibility and reduce protein adsorption on medical implants. The application of PMETAC as a coating on poly(ether ketone) substrates has been shown to significantly decrease fibrinogen adsorption, thereby minimizing implant overgrowth and enhancing the longevity of medical devices .
Summary Table of Applications
Application Area | Specific Use Case | Key Findings |
---|---|---|
Biomedical | Encapsulation of pancreatic islets | Extended insulin secretion (up to 90 days) |
Antimicrobial coatings | Effective against Candida spp. and bacteria | |
Environmental | Water treatment | Adsorption of dyes and heavy metals |
Material Science | Smart adhesives | Enhanced antibacterial properties |
Surface modification | Reduced protein adsorption on implants |
Properties
CAS No. |
15302-88-2 |
---|---|
Molecular Formula |
C5H14N+ |
Molecular Weight |
88.17 g/mol |
IUPAC Name |
ethyl(trimethyl)azanium |
InChI |
InChI=1S/C5H14N/c1-5-6(2,3)4/h5H2,1-4H3/q+1 |
InChI Key |
YOMFVLRTMZWACQ-UHFFFAOYSA-N |
SMILES |
CC[N+](C)(C)C |
Canonical SMILES |
CC[N+](C)(C)C |
Key on ui other cas no. |
15302-88-2 |
Related CAS |
2650-77-3 (bromide) 27697-51-4 (chloride) 30382-83-3 (hydroxide) 51-93-4 (iodide) |
Synonyms |
ethyltrimethylammonium TMEA chloride trimethylethylammonium trimethylethylammonium acetate trimethylethylammonium bromide trimethylethylammonium chloride trimethylethylammonium hydroxide trimethylethylammonium iodide trimethylethylammonium sulfite (1:1) |
Origin of Product |
United States |
Retrosynthesis Analysis
AI-Powered Synthesis Planning: Our tool employs the Template_relevance Pistachio, Template_relevance Bkms_metabolic, Template_relevance Pistachio_ringbreaker, Template_relevance Reaxys, Template_relevance Reaxys_biocatalysis model, leveraging a vast database of chemical reactions to predict feasible synthetic routes.
One-Step Synthesis Focus: Specifically designed for one-step synthesis, it provides concise and direct routes for your target compounds, streamlining the synthesis process.
Accurate Predictions: Utilizing the extensive PISTACHIO, BKMS_METABOLIC, PISTACHIO_RINGBREAKER, REAXYS, REAXYS_BIOCATALYSIS database, our tool offers high-accuracy predictions, reflecting the latest in chemical research and data.
Strategy Settings
Precursor scoring | Relevance Heuristic |
---|---|
Min. plausibility | 0.01 |
Model | Template_relevance |
Template Set | Pistachio/Bkms_metabolic/Pistachio_ringbreaker/Reaxys/Reaxys_biocatalysis |
Top-N result to add to graph | 6 |
Feasible Synthetic Routes
Disclaimer and Information on In-Vitro Research Products
Please be aware that all articles and product information presented on BenchChem are intended solely for informational purposes. The products available for purchase on BenchChem are specifically designed for in-vitro studies, which are conducted outside of living organisms. In-vitro studies, derived from the Latin term "in glass," involve experiments performed in controlled laboratory settings using cells or tissues. It is important to note that these products are not categorized as medicines or drugs, and they have not received approval from the FDA for the prevention, treatment, or cure of any medical condition, ailment, or disease. We must emphasize that any form of bodily introduction of these products into humans or animals is strictly prohibited by law. It is essential to adhere to these guidelines to ensure compliance with legal and ethical standards in research and experimentation.