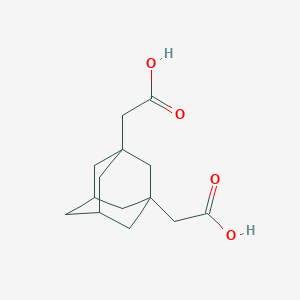
1,3-Adamantanediacetic acid
Overview
Description
1,3-Adamantanediacetic acid (H₂ADA, C₁₄H₂₀O₄, molecular weight: 252.31 g/mol) is a flexible alicyclic dicarboxylate ligand characterized by two acetic acid groups attached to the 1,3-positions of an adamantane backbone . Its unique structure imparts high thermal stability (melting point: 234–237°C) and moderate solubility in polar solvents like methanol . H₂ADA is widely employed in constructing metal-organic frameworks (MOFs) and coordination polymers (CPs), particularly with lanthanides (Ln), due to its ability to adopt diverse coordination modes. This flexibility enables the formation of 1D, 2D, and 3D architectures under varying synthetic conditions, as demonstrated in studies involving europium (Eu) and terbium (Tb) clusters for luminescent sensors .
Preparation Methods
Synthetic Routes and Reaction Conditions
1,3-Adamantanediacetic acid can be synthesized through several methods. One common approach involves the reaction of 1,3-dibromoadamantane with sodium cyanide to form 1,3-dicyanoadamantane, which is then hydrolyzed to yield this compound . The reaction conditions typically involve the use of solvents such as dimethylformamide and temperatures around 100°C.
Industrial Production Methods
Industrial production of this compound often involves the catalytic hydrogenation of 1,3-dicyanoadamantane in the presence of a palladium catalyst. This method is preferred due to its efficiency and scalability .
Chemical Reactions Analysis
Types of Reactions
1,3-Adamantanediacetic acid undergoes various chemical reactions, including:
Oxidation: It can be oxidized to form corresponding carboxylic acids.
Reduction: Reduction reactions can convert it into alcohols or other reduced forms.
Substitution: It can undergo substitution reactions where the carboxylic acid groups are replaced by other functional groups.
Common Reagents and Conditions
Oxidation: Common oxidizing agents include potassium permanganate and chromium trioxide.
Reduction: Reducing agents such as lithium aluminum hydride and sodium borohydride are often used.
Substitution: Reagents like thionyl chloride and phosphorus tribromide are used for substitution reactions.
Major Products Formed
The major products formed from these reactions include various derivatives of adamantane, such as adamantane-1,3-dicarboxylic acid and adamantane-1,3-diol .
Scientific Research Applications
1,3-Adamantanediacetic acid has a wide range of scientific research applications:
Chemistry: It is used as a building block in the synthesis of complex organic molecules and coordination polymers.
Biology: Its derivatives are studied for their potential antiviral and antitumor properties.
Industry: It is used in the production of polymers and other materials with unique properties.
Mechanism of Action
The mechanism of action of 1,3-adamantanediacetic acid and its derivatives involves their interaction with specific molecular targets. For example, derivatives of this compound have been shown to inhibit the replication of influenza A virus by targeting viral proteins and interfering with their function . The adamantane core provides a stable and rigid framework that enhances the compound’s binding affinity to its molecular targets .
Comparison with Similar Compounds
Structural and Functional Group Variations
1,3-Adamantanedicarboxylic Acid (H₂ADC)
- Substituents : H₂ADC features two carboxylic acid groups directly bonded to the adamantane core, unlike H₂ADA’s acetic acid arms.
- Coordination Behavior : The shorter carboxylic groups in H₂ADC restrict conformational flexibility compared to H₂ADA, leading to distinct Zn(II) coordination polymers. For example, [Zn(ADC)(bimh)]ₙ forms different topologies than [Zn(ADA)(bimh)]ₙ due to steric and electronic differences .
- Synthesis : H₂ADC is synthesized via the Koch–Haaf reaction, whereas H₂ADA derivatives are often prepared through hydrolysis and esterification .
Rigid Aromatic Ligands (H₃BTC, H₂NDC)
- Flexibility : Rigid aromatic linkers like 1,3,5-benzenetricarboxylic acid (H₃BTC) and 2,6-naphthalenedicarboxylic acid (H₂NDC) lack the conformational adaptability of H₂ADA.
- MOF Porosity : Carbons derived from H₃BTC- or H₂NDC-based MOFs exhibit higher surface areas (SBET: 920–2184 m²/g) compared to H₂ADA-derived carbons (SBET: 513 m²/g) due to ordered carbon sheet formation .
- Morphology : H₂ADA-based MOFs yield less-ordered 2D carbon sheets, whereas rigid ligands produce periodically arranged structures .
Adamantane Derivatives with Heterocyclic Groups
- Functionalization : Compounds like 3-(3-methyl-1,2,4-triazol-1-yl)-adamantane-1-carboxylic acid (mtrzadcH) incorporate nitrogen-rich heterocycles, enabling distinct coordination chemistry and applications in catalysis or bioactivity .
Coordination Chemistry and MOF Performance
Key Research Findings
Structural Adaptability : H₂ADA’s flexible coordination modes enable pH-dependent formation of Co(II) polymers, ranging from 1D chains to 2D networks .
Luminescent Efficiency : Tb-H₂ADA MOFs exhibit quantum yields up to 45%, outperforming many lanthanide complexes with rigid ligands .
Thermal Robustness : H₂ADA-based Ln-MOFs retain crystallinity up to 400°C, making them suitable for high-temperature applications .
Biological Activity
1,3-Adamantanediacetic acid (H2ADA) is a derivative of adamantane that has garnered attention for its diverse biological activities, particularly in antiviral and potential anticancer applications. This article delves into the compound's biological activity, mechanisms of action, and relevant research findings.
Chemical Structure and Properties
This compound is characterized by its unique adamantane structure, which contributes to its stability and biological interactions. The molecular formula is , and it features two carboxylic acid groups attached to a central adamantane framework.
Antiviral Activity:
this compound has demonstrated significant antiviral properties, particularly against the influenza A virus. The compound selectively inhibits viral replication by interfering with the virus's ability to replicate within host cells. This action is attributed to its structural similarity to other known antiviral agents, such as rimantadine, which disrupts ion transport in viral membranes by binding to the M2 protein .
Biochemical Pathways:
The compound's interaction with cellular pathways involves modulation of various biochemical processes. It has been shown to form complexes with metal ions like zinc and cadmium, which may enhance its biological effects . Additionally, H2ADA has been studied for its potential in drug development, particularly in the design of novel antiviral agents.
Antiviral Studies
A study highlighted the compound's effectiveness against influenza A virus strains. In vitro assays demonstrated that H2ADA inhibited viral replication in a dose-dependent manner. The mechanism involved blocking the M2 proton channel, thereby preventing the necessary acidification required for viral uncoating and replication .
Antitumor Potential
Recent investigations have explored the potential anticancer properties of this compound. In vitro studies indicated that derivatives of H2ADA exhibited cytotoxic effects on various cancer cell lines. These effects were linked to the compound's ability to induce apoptosis and inhibit cell proliferation .
Case Studies
Case Study 1: Influenza A Virus Inhibition
In a controlled laboratory setting, researchers treated human lung epithelial cells infected with influenza A with varying concentrations of this compound. Results showed a significant reduction in viral load compared to untreated controls, confirming its antiviral efficacy.
Case Study 2: Cytotoxicity in Cancer Cells
A separate study assessed the cytotoxic effects of H2ADA on human breast cancer cells (MCF-7). The results indicated that treatment with H2ADA led to increased levels of active caspase-3, a marker of apoptosis, suggesting that the compound may induce programmed cell death in cancerous cells .
Comparative Data Table
Property | This compound | Rimantadine |
---|---|---|
Molecular Formula | C14H20O4 | C12H21N |
Mechanism of Action | Inhibits influenza A replication | M2 protein inhibitor |
Biological Activity | Antiviral, Antitumor | Antiviral |
Cytotoxicity | Yes (in cancer cells) | Limited |
Q & A
Q. Basic Synthesis: What are the standard methodologies for synthesizing H₂ADA-based coordination polymers?
Answer:
H₂ADA is typically employed in hydrothermal/solvothermal synthesis to construct metal-organic frameworks (MOFs) or coordination polymers. Key steps include:
- Reagents : Combine H₂ADA with metal salts (e.g., Co(NO₃)₂, UO₂(NO₃)₂·6H₂O) and auxiliary ligands (e.g., 4,4′-bipyridine) in aqueous or mixed solvent systems .
- Conditions : Heat at 140°C under autogenous pressure in sealed vessels for 24–72 hours to promote crystallization .
- pH Control : Adjust pH (e.g., 5–7) to modulate ligand deprotonation and metal-ligand coordination modes, yielding diverse architectures (1D chains, 2D sheets, 3D frameworks) .
Q. Structural Characterization: What techniques are critical for analyzing H₂ADA-based complexes?
Answer:
- Single-Crystal X-Ray Diffraction : Resolves atomic-level structures, revealing coordination geometries (e.g., uranyl hexagonal bipyramidal geometry in uranium complexes) .
- FT-IR Spectroscopy : Identifies ligand deprotonation (shift in ν(COO⁻) stretches from ~1700 cm⁻¹ to ~1550–1400 cm⁻¹) and metal-ligand binding .
- Powder XRD : Confirms phase purity and matches simulated patterns from single-crystal data .
- Thermogravimetric Analysis (TGA) : Assesses thermal stability; H₂ADA-based MOFs often degrade above 300°C .
Q. pH Influence: How does pH affect the structural diversity of H₂ADA coordination polymers?
Answer:
pH modulates ligand protonation states and metal hydrolysis:
- Low pH (≤5) : H₂ADA remains partially protonated (HADA⁻), favoring monodentate coordination. Example: [Co(HADA)₂(bpp)]ₙ forms 1D chains via HADA⁻ and bpp linkers .
- Neutral pH (6–7) : Full deprotonation (ADA²⁻) enables polydentate binding. Example: [Co(ADA)(bpp)(CH₃OH)]ₙ forms 2D sheets through ADA²⁻ bridging .
- Methodology : Use dilute HNO₃ or NaOH to adjust pH pre-synthesis; monitor via in-situ pH probes .
Q. Advanced: How do counterions influence the topology of uranyl-H₂ADA complexes?
Answer:
Counterions template distinct architectures by competing for coordination sites:
- Nitrate (NO₃⁻) : Acts as a terminal ligand, enabling simple chains (e.g., [PPh₃Me][UO₂(ADC)(NO₃)]) .
- Phosphate (PO₄³⁻) or Formate (HCOO⁻) : Bridge uranyl centers, forming 2D/3D networks (e.g., [(UO₂)₂(ADA)₂Ni(cyclam)] with Ni²⁺-formate bridges) .
- Analysis : Compare luminescence spectra and X-ray structures to correlate counterion effects with topology .
Q. Advanced: What methodologies enable luminescence tuning in H₂ADA-based Ln-MOFs?
Answer:
- Lanthanide Doping : Incorporate Eu³⁺ or Tb³⁺ into H₂ADA frameworks; ligand-to-metal energy transfer (LMET) enhances emission .
- Sensing Applications : Monitor luminescence quenching upon analyte binding (e.g., antibiotics detected via Förster resonance energy transfer (FRET)) .
- Quantum Yield Measurement : Use integrating sphere setups; reported yields for H₂ADA-Eu³⁺ MOFs are low (<5%) due to non-radiative decay .
Q. Safety: What precautions are required when synthesizing H₂ADA complexes with radioactive elements?
Answer:
- Containment : Use fume hoods and lead shielding for uranium-containing reactions .
- Waste Handling : Store radioactive waste in labeled, sealed containers; follow institutional protocols for disposal .
- Personal Protection : Wear lab coats, gloves, and dosimeters during handling .
Q. Data Contradictions: Why do similar synthetic conditions yield divergent structures?
Answer:
Minor variations (e.g., solvent polarity, cooling rates) can alter nucleation:
- Case Study : Hydrothermal synthesis with H₂ADA and Co²⁺ yields 1D chains ([Co(HADA)₂(4,4′-bipy)(H₂O)₂]ₙ) or 2D sheets ([Co(ADA)(4,4′-bipy)₀.₅]ₙ) depending on methanol/water ratios .
- Resolution : Replicate experiments with strict control of variables; use high-throughput screening to map phase diagrams .
Q. Thermodynamic Stability: How to assess the stability of H₂ADA-MOFs?
Answer:
- TGA-DSC : Determine decomposition temperatures (e.g., H₂ADA-Co polymers stable up to 250°C) .
- Solvent Stability : Soak crystals in water/organic solvents for 24h; analyze PXRD post-treatment to check framework integrity .
Q. Advanced: How does H₂ADA compare to other adamantane ligands in tuning supramolecular assemblies?
Answer:
- Flexibility : H₂ADA’s CH₂ spacers allow conformational flexibility vs. rigid 1,3-adamantanedicarboxylic acid (H₂ADC), enabling adaptive coordination (e.g., trough-like uranyl assemblies) .
- Charge Density : H₂ADA’s lower charge density (vs. H₂ADC) reduces interlayer electrostatic repulsion, facilitating 3D frameworks .
Q. Charge Tuning: Can H₂ADA modulate micelle core-unit charges in coacervate systems?
Answer:
Properties
IUPAC Name |
2-[3-(carboxymethyl)-1-adamantyl]acetic acid | |
---|---|---|
Source | PubChem | |
URL | https://pubchem.ncbi.nlm.nih.gov | |
Description | Data deposited in or computed by PubChem | |
InChI |
InChI=1S/C14H20O4/c15-11(16)6-13-2-9-1-10(4-13)5-14(3-9,8-13)7-12(17)18/h9-10H,1-8H2,(H,15,16)(H,17,18) | |
Source | PubChem | |
URL | https://pubchem.ncbi.nlm.nih.gov | |
Description | Data deposited in or computed by PubChem | |
InChI Key |
UTENGZNBNPABQE-UHFFFAOYSA-N | |
Source | PubChem | |
URL | https://pubchem.ncbi.nlm.nih.gov | |
Description | Data deposited in or computed by PubChem | |
Canonical SMILES |
C1C2CC3(CC1CC(C2)(C3)CC(=O)O)CC(=O)O | |
Source | PubChem | |
URL | https://pubchem.ncbi.nlm.nih.gov | |
Description | Data deposited in or computed by PubChem | |
Molecular Formula |
C14H20O4 | |
Source | PubChem | |
URL | https://pubchem.ncbi.nlm.nih.gov | |
Description | Data deposited in or computed by PubChem | |
DSSTOX Substance ID |
DTXSID30170366 | |
Record name | Tricyclo(3.3.1.13,7)dec-1,3-diyldi(acetic acid) | |
Source | EPA DSSTox | |
URL | https://comptox.epa.gov/dashboard/DTXSID30170366 | |
Description | DSSTox provides a high quality public chemistry resource for supporting improved predictive toxicology. | |
Molecular Weight |
252.31 g/mol | |
Source | PubChem | |
URL | https://pubchem.ncbi.nlm.nih.gov | |
Description | Data deposited in or computed by PubChem | |
CAS No. |
17768-28-4 | |
Record name | 1,3-Adamantanediacetic acid | |
Source | CAS Common Chemistry | |
URL | https://commonchemistry.cas.org/detail?cas_rn=17768-28-4 | |
Description | CAS Common Chemistry is an open community resource for accessing chemical information. Nearly 500,000 chemical substances from CAS REGISTRY cover areas of community interest, including common and frequently regulated chemicals, and those relevant to high school and undergraduate chemistry classes. This chemical information, curated by our expert scientists, is provided in alignment with our mission as a division of the American Chemical Society. | |
Explanation | The data from CAS Common Chemistry is provided under a CC-BY-NC 4.0 license, unless otherwise stated. | |
Record name | Tricyclo(3.3.1.13,7)dec-1,3-diyldi(acetic acid) | |
Source | ChemIDplus | |
URL | https://pubchem.ncbi.nlm.nih.gov/substance/?source=chemidplus&sourceid=0017768284 | |
Description | ChemIDplus is a free, web search system that provides access to the structure and nomenclature authority files used for the identification of chemical substances cited in National Library of Medicine (NLM) databases, including the TOXNET system. | |
Record name | Tricyclo(3.3.1.13,7)dec-1,3-diyldi(acetic acid) | |
Source | EPA DSSTox | |
URL | https://comptox.epa.gov/dashboard/DTXSID30170366 | |
Description | DSSTox provides a high quality public chemistry resource for supporting improved predictive toxicology. | |
Record name | Tricyclo[3.3.1.13,7]dec-1,3-diyldi(acetic acid) | |
Source | European Chemicals Agency (ECHA) | |
URL | https://echa.europa.eu/substance-information/-/substanceinfo/100.037.940 | |
Description | The European Chemicals Agency (ECHA) is an agency of the European Union which is the driving force among regulatory authorities in implementing the EU's groundbreaking chemicals legislation for the benefit of human health and the environment as well as for innovation and competitiveness. | |
Explanation | Use of the information, documents and data from the ECHA website is subject to the terms and conditions of this Legal Notice, and subject to other binding limitations provided for under applicable law, the information, documents and data made available on the ECHA website may be reproduced, distributed and/or used, totally or in part, for non-commercial purposes provided that ECHA is acknowledged as the source: "Source: European Chemicals Agency, http://echa.europa.eu/". Such acknowledgement must be included in each copy of the material. ECHA permits and encourages organisations and individuals to create links to the ECHA website under the following cumulative conditions: Links can only be made to webpages that provide a link to the Legal Notice page. | |
Retrosynthesis Analysis
AI-Powered Synthesis Planning: Our tool employs the Template_relevance Pistachio, Template_relevance Bkms_metabolic, Template_relevance Pistachio_ringbreaker, Template_relevance Reaxys, Template_relevance Reaxys_biocatalysis model, leveraging a vast database of chemical reactions to predict feasible synthetic routes.
One-Step Synthesis Focus: Specifically designed for one-step synthesis, it provides concise and direct routes for your target compounds, streamlining the synthesis process.
Accurate Predictions: Utilizing the extensive PISTACHIO, BKMS_METABOLIC, PISTACHIO_RINGBREAKER, REAXYS, REAXYS_BIOCATALYSIS database, our tool offers high-accuracy predictions, reflecting the latest in chemical research and data.
Strategy Settings
Precursor scoring | Relevance Heuristic |
---|---|
Min. plausibility | 0.01 |
Model | Template_relevance |
Template Set | Pistachio/Bkms_metabolic/Pistachio_ringbreaker/Reaxys/Reaxys_biocatalysis |
Top-N result to add to graph | 6 |
Feasible Synthetic Routes
Disclaimer and Information on In-Vitro Research Products
Please be aware that all articles and product information presented on BenchChem are intended solely for informational purposes. The products available for purchase on BenchChem are specifically designed for in-vitro studies, which are conducted outside of living organisms. In-vitro studies, derived from the Latin term "in glass," involve experiments performed in controlled laboratory settings using cells or tissues. It is important to note that these products are not categorized as medicines or drugs, and they have not received approval from the FDA for the prevention, treatment, or cure of any medical condition, ailment, or disease. We must emphasize that any form of bodily introduction of these products into humans or animals is strictly prohibited by law. It is essential to adhere to these guidelines to ensure compliance with legal and ethical standards in research and experimentation.