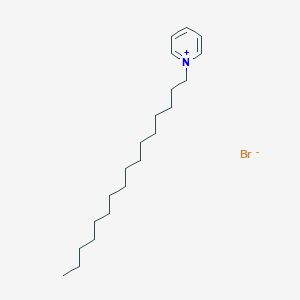
Cetylpyridinium bromide
Overview
Description
Cetylpyridinium is a quaternary ammonium with broad-spectrum antiseptic properties . Its salt form, cetylpyridinium bromide, is typically found as an active ingredient in mouthwashes, toothpastes, lozenges, throat sprays, breath sprays, and nasal sprays . The molecular formula is C21H38BrN .
Synthesis Analysis
Cetylpyridinium bromide can be synthesized by a cationic interchange process . It has been used as a template in the synthesis of mesoporous aluminosilicates . The use of cetylpyridinium bromide as a template led to an increase in aluminosilicates’ specific surface areas and mesopore volumes compared to the use of cetylpyridinium chloride .Molecular Structure Analysis
The presence of cetylpyridinium bromide in the clay structure was evidenced by X-ray diffraction (XRD), Fourier transform infrared (FTIR) spectroscopy, and thermogravimetric analysis (TGA) . The molecular weight is 384.44 (anhydrous basis) .Physical And Chemical Properties Analysis
The physical and chemical properties of cetylpyridinium bromide have been studied using techniques such as XRD, FTIR, differential scanning calorimetry, TGA, optical and mechanical analyses . The critical micelle concentration (CMC) and the extent of counter ion bound (b) were evaluated for the cetylpyridinium bromide system .Scientific Research Applications
1. Extraction and Preconcentration of Ionic Species CPB has been used in the extraction and preconcentration of ionic species. It plays a significant role in the polymerization reaction .
Polymerization
CPB is used in polymerization processes. It helps in the formation of polymers by acting as a catalyst or a reactant .
Protein Folding
CPB has been explored for its use in protein folding. It can interact with proteins and influence their folding process, which is crucial in biological functions .
Enzyme Studies
CPB is used in enzyme studies. It can interact with enzymes and affect their activity, providing valuable insights into enzyme functions .
Gene Delivery
CPB has been used in gene delivery. It can bind to genetic material and facilitate its delivery into cells, which is essential in genetic engineering and therapy .
Pharmaceuticals and Drug Delivery
CPB is used in pharmaceuticals as a drug delivery tool. It can encapsulate drugs and enhance their delivery to the target site .
Disinfectant and Germicide
CPB is used as a disinfectant and germicide. It has antimicrobial properties and is used in products like mouthwashes .
Removal of Heavy Metals
CPB has been used in the removal of heavy metals from water. For example, it has been used in the construction of composite beads for the efficient adsorptive removal of Cr (VI) ions .
Mechanism of Action
Target of Action
Cetylpyridinium bromide is a quaternary ammonium compound with broad-spectrum antiseptic properties . Its primary targets are microbial cell surfaces, particularly those of gram-positive pathogens and yeasts . The compound interacts with these cell surfaces and can even integrate into the bacterial cytoplasmic membrane .
Mode of Action
The mode of action of cetylpyridinium bromide involves the positively charged hydrophilic region of its molecules. This allows the compound to interact with microbial cell surfaces and even integrate into the bacterial cytoplasmic membrane . This interaction disrupts the cell membrane, leading to leakage of intracellular contents and eventually cell death .
Biochemical Pathways
Cetylpyridinium bromide affects the biochemical pathways related to cell membrane integrity. By integrating into the bacterial cytoplasmic membrane, it disrupts normal cell function, leading to cell death
Result of Action
The result of cetylpyridinium bromide’s action is the reduction of bacterial load at the site of application. It achieves this by disrupting the cell membranes of bacteria, leading to cell death . This results in an antiseptic activity and protective action against dental plaque and reducing gingivitis .
Safety and Hazards
Future Directions
properties
IUPAC Name |
1-hexadecylpyridin-1-ium;bromide | |
---|---|---|
Source | PubChem | |
URL | https://pubchem.ncbi.nlm.nih.gov | |
Description | Data deposited in or computed by PubChem | |
InChI |
InChI=1S/C21H38N.BrH/c1-2-3-4-5-6-7-8-9-10-11-12-13-14-16-19-22-20-17-15-18-21-22;/h15,17-18,20-21H,2-14,16,19H2,1H3;1H/q+1;/p-1 | |
Source | PubChem | |
URL | https://pubchem.ncbi.nlm.nih.gov | |
Description | Data deposited in or computed by PubChem | |
InChI Key |
DVBJBNKEBPCGSY-UHFFFAOYSA-M | |
Source | PubChem | |
URL | https://pubchem.ncbi.nlm.nih.gov | |
Description | Data deposited in or computed by PubChem | |
Canonical SMILES |
CCCCCCCCCCCCCCCC[N+]1=CC=CC=C1.[Br-] | |
Source | PubChem | |
URL | https://pubchem.ncbi.nlm.nih.gov | |
Description | Data deposited in or computed by PubChem | |
Molecular Formula |
C21H38BrN | |
Source | PubChem | |
URL | https://pubchem.ncbi.nlm.nih.gov | |
Description | Data deposited in or computed by PubChem | |
DSSTOX Substance ID |
DTXSID3033307 | |
Record name | Cetylpyridinium bromide | |
Source | EPA DSSTox | |
URL | https://comptox.epa.gov/dashboard/DTXSID3033307 | |
Description | DSSTox provides a high quality public chemistry resource for supporting improved predictive toxicology. | |
Molecular Weight |
384.4 g/mol | |
Source | PubChem | |
URL | https://pubchem.ncbi.nlm.nih.gov | |
Description | Data deposited in or computed by PubChem | |
Physical Description |
Cream-colored solid; [HSDB] White powder; [Acros Organics MSDS] | |
Record name | Cetylpyridinium bromide | |
Source | Haz-Map, Information on Hazardous Chemicals and Occupational Diseases | |
URL | https://haz-map.com/Agents/12878 | |
Description | Haz-Map® is an occupational health database designed for health and safety professionals and for consumers seeking information about the adverse effects of workplace exposures to chemical and biological agents. | |
Explanation | Copyright (c) 2022 Haz-Map(R). All rights reserved. Unless otherwise indicated, all materials from Haz-Map are copyrighted by Haz-Map(R). No part of these materials, either text or image may be used for any purpose other than for personal use. Therefore, reproduction, modification, storage in a retrieval system or retransmission, in any form or by any means, electronic, mechanical or otherwise, for reasons other than personal use, is strictly prohibited without prior written permission. | |
Solubility |
Soluble in acetone, ethanol, and chloroform | |
Record name | CETYLPYRIDINIUM BROMIDE | |
Source | Hazardous Substances Data Bank (HSDB) | |
URL | https://pubchem.ncbi.nlm.nih.gov/source/hsdb/6859 | |
Description | The Hazardous Substances Data Bank (HSDB) is a toxicology database that focuses on the toxicology of potentially hazardous chemicals. It provides information on human exposure, industrial hygiene, emergency handling procedures, environmental fate, regulatory requirements, nanomaterials, and related areas. The information in HSDB has been assessed by a Scientific Review Panel. | |
Product Name |
Cetylpyridinium bromide | |
Color/Form |
Cream-colored, waxy solid | |
CAS RN |
140-72-7 | |
Record name | Cetylpyridinium bromide | |
Source | CAS Common Chemistry | |
URL | https://commonchemistry.cas.org/detail?cas_rn=140-72-7 | |
Description | CAS Common Chemistry is an open community resource for accessing chemical information. Nearly 500,000 chemical substances from CAS REGISTRY cover areas of community interest, including common and frequently regulated chemicals, and those relevant to high school and undergraduate chemistry classes. This chemical information, curated by our expert scientists, is provided in alignment with our mission as a division of the American Chemical Society. | |
Explanation | The data from CAS Common Chemistry is provided under a CC-BY-NC 4.0 license, unless otherwise stated. | |
Record name | Cetylpyridinium bromide | |
Source | ChemIDplus | |
URL | https://pubchem.ncbi.nlm.nih.gov/substance/?source=chemidplus&sourceid=0000140727 | |
Description | ChemIDplus is a free, web search system that provides access to the structure and nomenclature authority files used for the identification of chemical substances cited in National Library of Medicine (NLM) databases, including the TOXNET system. | |
Record name | CETYLPYRIDINIUM BROMIDE | |
Source | DTP/NCI | |
URL | https://dtp.cancer.gov/dtpstandard/servlet/dwindex?searchtype=NSC&outputformat=html&searchlist=8495 | |
Description | The NCI Development Therapeutics Program (DTP) provides services and resources to the academic and private-sector research communities worldwide to facilitate the discovery and development of new cancer therapeutic agents. | |
Explanation | Unless otherwise indicated, all text within NCI products is free of copyright and may be reused without our permission. Credit the National Cancer Institute as the source. | |
Record name | Pyridinium, 1-hexadecyl-, bromide (1:1) | |
Source | EPA Chemicals under the TSCA | |
URL | https://www.epa.gov/chemicals-under-tsca | |
Description | EPA Chemicals under the Toxic Substances Control Act (TSCA) collection contains information on chemicals and their regulations under TSCA, including non-confidential content from the TSCA Chemical Substance Inventory and Chemical Data Reporting. | |
Record name | Cetylpyridinium bromide | |
Source | EPA DSSTox | |
URL | https://comptox.epa.gov/dashboard/DTXSID3033307 | |
Description | DSSTox provides a high quality public chemistry resource for supporting improved predictive toxicology. | |
Record name | Cetylpyridinium bromide | |
Source | European Chemicals Agency (ECHA) | |
URL | https://echa.europa.eu/substance-information/-/substanceinfo/100.004.936 | |
Description | The European Chemicals Agency (ECHA) is an agency of the European Union which is the driving force among regulatory authorities in implementing the EU's groundbreaking chemicals legislation for the benefit of human health and the environment as well as for innovation and competitiveness. | |
Explanation | Use of the information, documents and data from the ECHA website is subject to the terms and conditions of this Legal Notice, and subject to other binding limitations provided for under applicable law, the information, documents and data made available on the ECHA website may be reproduced, distributed and/or used, totally or in part, for non-commercial purposes provided that ECHA is acknowledged as the source: "Source: European Chemicals Agency, http://echa.europa.eu/". Such acknowledgement must be included in each copy of the material. ECHA permits and encourages organisations and individuals to create links to the ECHA website under the following cumulative conditions: Links can only be made to webpages that provide a link to the Legal Notice page. | |
Record name | CETYLPYRIDINIUM BROMIDE | |
Source | FDA Global Substance Registration System (GSRS) | |
URL | https://gsrs.ncats.nih.gov/ginas/app/beta/substances/O77BKZ14DE | |
Description | The FDA Global Substance Registration System (GSRS) enables the efficient and accurate exchange of information on what substances are in regulated products. Instead of relying on names, which vary across regulatory domains, countries, and regions, the GSRS knowledge base makes it possible for substances to be defined by standardized, scientific descriptions. | |
Explanation | Unless otherwise noted, the contents of the FDA website (www.fda.gov), both text and graphics, are not copyrighted. They are in the public domain and may be republished, reprinted and otherwise used freely by anyone without the need to obtain permission from FDA. Credit to the U.S. Food and Drug Administration as the source is appreciated but not required. | |
Record name | CETYLPYRIDINIUM BROMIDE | |
Source | Hazardous Substances Data Bank (HSDB) | |
URL | https://pubchem.ncbi.nlm.nih.gov/source/hsdb/6859 | |
Description | The Hazardous Substances Data Bank (HSDB) is a toxicology database that focuses on the toxicology of potentially hazardous chemicals. It provides information on human exposure, industrial hygiene, emergency handling procedures, environmental fate, regulatory requirements, nanomaterials, and related areas. The information in HSDB has been assessed by a Scientific Review Panel. | |
Melting Point |
64.5 °C | |
Record name | CETYLPYRIDINIUM BROMIDE | |
Source | Hazardous Substances Data Bank (HSDB) | |
URL | https://pubchem.ncbi.nlm.nih.gov/source/hsdb/6859 | |
Description | The Hazardous Substances Data Bank (HSDB) is a toxicology database that focuses on the toxicology of potentially hazardous chemicals. It provides information on human exposure, industrial hygiene, emergency handling procedures, environmental fate, regulatory requirements, nanomaterials, and related areas. The information in HSDB has been assessed by a Scientific Review Panel. | |
Retrosynthesis Analysis
AI-Powered Synthesis Planning: Our tool employs the Template_relevance Pistachio, Template_relevance Bkms_metabolic, Template_relevance Pistachio_ringbreaker, Template_relevance Reaxys, Template_relevance Reaxys_biocatalysis model, leveraging a vast database of chemical reactions to predict feasible synthetic routes.
One-Step Synthesis Focus: Specifically designed for one-step synthesis, it provides concise and direct routes for your target compounds, streamlining the synthesis process.
Accurate Predictions: Utilizing the extensive PISTACHIO, BKMS_METABOLIC, PISTACHIO_RINGBREAKER, REAXYS, REAXYS_BIOCATALYSIS database, our tool offers high-accuracy predictions, reflecting the latest in chemical research and data.
Strategy Settings
Precursor scoring | Relevance Heuristic |
---|---|
Min. plausibility | 0.01 |
Model | Template_relevance |
Template Set | Pistachio/Bkms_metabolic/Pistachio_ringbreaker/Reaxys/Reaxys_biocatalysis |
Top-N result to add to graph | 6 |
Feasible Synthetic Routes
Disclaimer and Information on In-Vitro Research Products
Please be aware that all articles and product information presented on BenchChem are intended solely for informational purposes. The products available for purchase on BenchChem are specifically designed for in-vitro studies, which are conducted outside of living organisms. In-vitro studies, derived from the Latin term "in glass," involve experiments performed in controlled laboratory settings using cells or tissues. It is important to note that these products are not categorized as medicines or drugs, and they have not received approval from the FDA for the prevention, treatment, or cure of any medical condition, ailment, or disease. We must emphasize that any form of bodily introduction of these products into humans or animals is strictly prohibited by law. It is essential to adhere to these guidelines to ensure compliance with legal and ethical standards in research and experimentation.