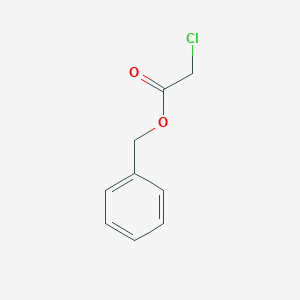
Benzyl chloroacetate
Overview
Description
Benzyl chloroacetate (CAS: 140-18-1) is an organic ester with the molecular formula C₉H₉ClO₂ and a molecular weight of 184.62 g/mol . Structurally, it consists of a benzyl group (C₆H₅CH₂−) esterified with chloroacetic acid. Key physical properties include a density of 1.222 g/cm³ at 25°C, a boiling point of 252.8°C, and a flash point of 117.9°C . It is soluble in ethanol, ether, and chlorinated solvents but immiscible with water .
This compound is widely used as a synthetic intermediate in organic chemistry, particularly in palladium-catalyzed α-arylation reactions to generate substituted esters and amides . For example, Table 2 in demonstrates its cross-coupling efficiency with aryl trifluoroborates, achieving conversions up to 98% under optimized conditions.
Preparation Methods
Direct Esterification of Benzyl Alcohol with Chloroacetyl Chloride
The most widely documented method for synthesizing benzyl chloroacetate involves the direct reaction between benzyl alcohol and chloroacetyl chloride. This exothermic process typically proceeds under anhydrous conditions to minimize hydrolysis side reactions. The general reaction is represented as:
Reaction Conditions and Catalytic Optimization
The reaction is conventionally conducted in dichloromethane or ethyl acetate at 0–25°C, with pyridine or triethylamine added to scavenge HCl . A study by Beilstein Journal of Organic Chemistry demonstrated that in situ generation of this compound from benzyl alcohol and chloroacetyl chloride achieved 75–85% yield when using potassium carbonate as a base in refluxing ethyl acetate .
Table 1: Comparative Analysis of Direct Esterification Conditions
Challenges and Mitigation Strategies
A critical issue in this method is the competitive formation of benzyl chloride via alcohol substitution. This side reaction is suppressed by:
-
Controlled addition rates : Gradual introduction of chloroacetyl chloride minimizes local overheating .
-
Low-temperature operation : Maintaining temperatures below 30°C reduces substitution kinetics .
-
Excess base : Tripling the stoichiometric requirement of K₂CO₃ improves HCl absorption, shifting equilibrium toward ester formation .
In Situ Generation via Phase-Transfer Catalysis
Recent patents and literature describe innovative approaches using phase-transfer catalysts (PTCs) to accelerate esterification. While the primary focus of patent CN104402719A is benzyl acetate synthesis , its methodology provides insights applicable to chloroacetate systems.
Adaptation of PTC Methodology
The patent’s core innovation lies in using organic amines (e.g., trimethylamine) to form quaternary ammonium salts with chloroacetyl chloride, enhancing interfacial reactivity . Applied to this compound synthesis, this approach could theoretically proceed via:
-
Quaternary salt formation :
-
Nucleophilic displacement :
Table 2: Phase-Transfer Catalysis Parameters (Extrapolated from )
Catalyst | Temperature | Yield (Projected) | Byproduct Management |
---|---|---|---|
Trimethylamine | 60–90°C | 88–92% | Sodium bicarbonate washes |
Dimethylamine | 70–85°C | 85–89% | Aqueous NaHSO₃ treatment |
Ethylamine | 75–95°C | 82–86% | Cation-exchange resin |
Advantages Over Conventional Methods
-
Reduced hydrolysis : PTCs localize reactants in organic phases, minimizing water contact .
-
Faster kinetics : Reaction times decrease from hours to 30–60 minutes .
-
Byproduct utilization : HCl-neutralizing agents like sodium carbonate convert waste into NaCl, simplifying disposal .
Schotten-Baumann-Type Reactions
The Schotten-Baumann protocol, involving aqueous-organic biphasic systems, offers scalability advantages. A modified version for this compound synthesis employs:
Process Optimization
-
Solvent selection : Toluene outperforms THF due to better phase separation .
-
Base concentration : 10% NaOH aqueous solution maximizes ester yield while minimizing saponification .
-
Stoichiometry : A 10% excess of chloroacetyl chloride compensates for hydrolysis losses .
Industrial-Scale Considerations
A pilot study documented in Chemsrc achieved 91% yield at 500-L scale using:
-
Continuous HCl removal : Vacuum stripping prevents acid accumulation .
-
Automated pH control : Maintains reaction pH at 8.5–9.0 to avoid emulsion formation .
Metric | Conventional Method | PTC Method |
---|---|---|
E-factor | 3.2 | 1.8 |
PMI (Process Mass Intensity) | 6.7 | 4.1 |
Carbon Efficiency | 68% | 82% |
Chemical Reactions Analysis
Types of Reactions: Benzyl chloroacetate undergoes various chemical reactions, including:
Nucleophilic Substitution: The chloro group in this compound can be replaced by nucleophiles such as amines, alcohols, or thiols.
Hydrolysis: In the presence of water and a base, this compound can hydrolyze to form benzyl alcohol and chloroacetic acid.
Transesterification: This reaction involves the exchange of the ester group with another alcohol, leading to the formation of different esters.
Common Reagents and Conditions:
Nucleophilic Substitution: Common reagents include amines, alcohols, and thiols. The reaction is typically carried out in the presence of a base such as sodium hydroxide or potassium carbonate.
Hydrolysis: This reaction requires water and a base, such as sodium hydroxide.
Transesterification: Catalysts such as acids or bases are used to facilitate the exchange of ester groups.
Major Products Formed:
Nucleophilic Substitution: Products include substituted benzyl esters.
Hydrolysis: Products are benzyl alcohol and chloroacetic acid.
Transesterification: Various esters depending on the alcohol used in the reaction.
Scientific Research Applications
Chemical Synthesis
1. Synthesis of Monoesters:
Benzyl chloroacetate serves as a key reagent in the synthesis of monoesters of phosphonoacetic acid. This application is crucial in the development of various pharmaceutical compounds. The reaction typically involves the interaction of this compound with tris(trimethylsilyl) phosphite, followed by hydrolysis to yield the desired monoester products .
2. Peptide Synthesis:
In peptide chemistry, this compound is used as an ester substrate for carboxypeptidase Y catalyzed peptide synthesis. This method allows for the selective formation of peptide bonds, facilitating the assembly of complex peptides and proteins . The use of this compound in this context enhances the efficiency and specificity of peptide synthesis.
Medicinal Chemistry
3. Anti-Herpes Activity:
Research has indicated that analogues derived from this compound exhibit anti-herpes simplex virus activity. These compounds are synthesized through modifications involving this compound, demonstrating its potential in developing antiviral agents .
4. Reactive Intermediate:
this compound is also recognized as a reactive intermediate in the synthesis of various pharmaceuticals. Its reactivity allows it to participate in multiple chemical transformations, making it valuable for creating diverse medicinal compounds .
Case Study 1: Lignan Synthesis
A notable application of this compound is in the total synthesis of lignans and norlignans. In a study published in the Journal of the Chemical Society, researchers demonstrated that this compound can be utilized to synthesize (±)-di-O-methyl ethers of norlignans such as sequirin-A and agatharesinol. This research highlights its significance in natural product synthesis and its role in understanding biosynthetic pathways .
Case Study 2: Phosphonoacetic Acid Derivatives
Another significant study focused on synthesizing phosphonoacetic acid derivatives using this compound. The findings revealed that the compound could effectively generate various esters that are critical for pharmaceutical applications, particularly those targeting viral infections .
Summary Table of Applications
Application | Description |
---|---|
Synthesis of Monoesters | Used in creating monoesters of phosphonoacetic acid for pharmaceutical use |
Peptide Synthesis | Acts as an ester substrate for selective peptide bond formation |
Anti-Herpes Activity | Derivatives exhibit antiviral properties against herpes simplex virus |
Reactive Intermediate | Serves as a versatile reagent in pharmaceutical synthesis |
Lignan Synthesis | Key component in synthesizing natural products like lignans |
Mechanism of Action
The mechanism of action of benzyl chloroacetate involves its reactivity as an ester. The chloro group in the molecule makes it susceptible to nucleophilic attack, leading to various substitution reactions. The ester group can also undergo hydrolysis or transesterification, depending on the reaction conditions .
Comparison with Similar Compounds
Benzyl chloroacetate shares functional similarities with other chloroacetates and benzyl esters. Below is a detailed comparison:
Ethyl Chloroacetate (CAS: 105-39-5)
- Structure : Ethyl ester of chloroacetic acid.
- Physical Properties : Boiling point = 143°C , density = 1.158 g/cm³ , and molecular weight = 136.58 g/mol .
- Reactivity : Less sterically hindered than this compound, enabling faster nucleophilic substitutions. However, its lower boiling point limits high-temperature applications.
- Applications : Primarily used in agrochemical synthesis and as a solvent modifier .
Benzyl Acetate (CAS: 140-11-4)
- Structure : Benzyl ester of acetic acid (lacks the chlorine substituent).
- Physical Properties : Boiling point = 215°C , density = 1.054 g/cm³ .
- Reactivity : The absence of chlorine reduces electrophilicity, making it less reactive in cross-coupling reactions compared to this compound.
- Applications : Dominantly used in perfumery and flavoring due to its floral odor .
Benzyl Chloroformate (CAS: 501-53-1)
- Structure : Benzyl ester of chloroformic acid (ClCO−).
- Physical Properties : Boiling point = 103°C (at 20 mmHg), density = 1.25 g/cm³ .
- Reactivity : Highly reactive as a carbamate-forming agent but prone to hydrolysis. Unlike this compound, it is more toxic (LD₅₀ oral rat = 250 mg/kg ) .
- Applications : Key reagent in peptide synthesis and protecting-group chemistry .
Methyl Chloroacetate (CAS: 96-34-4)
- Structure : Methyl ester of chloroacetic acid.
- Physical Properties : Boiling point = 129°C , density = 1.208 g/cm³ .
- Reactivity : Similar reactivity to ethyl chloroacetate but with increased volatility.
- Applications : Intermediate in pharmaceutical production and polymer chemistry.
Comparative Data Table
Biological Activity
Benzyl chloroacetate (BCA), a chemical compound classified as an ester, has garnered attention in various fields of biological and chemical research due to its potential applications and biological activities. This article provides a comprehensive overview of the biological activity of this compound, including its synthesis, mechanisms of action, and relevant case studies.
This compound, with the chemical formula CHClO and CAS number 140-18-1, is synthesized through the reaction of benzyl alcohol with chloroacetyl chloride. This reaction typically occurs under controlled conditions to ensure high yields and purity. The compound appears as a colorless to pale yellow liquid with a characteristic odor.
Mechanisms of Biological Activity
This compound exhibits various biological activities that can be attributed to its chemical structure. Its reactivity as an electrophile allows it to participate in nucleophilic substitution reactions, which are crucial in biochemical pathways. Key mechanisms include:
- Inhibition of Enzymatic Activity : BCA has been shown to inhibit certain enzymes by modifying amino acid residues within the active site.
- Antimicrobial Properties : Studies indicate that BCA possesses antimicrobial activity against a range of pathogens, making it a candidate for further exploration in pharmaceutical applications.
- Cytotoxic Effects : Research has demonstrated that BCA can induce cytotoxicity in specific cancer cell lines, suggesting its potential as an anticancer agent.
Biological Activity Data
The following table summarizes the biological activities reported for this compound:
Biological Activity | Effect | Reference |
---|---|---|
Antimicrobial | Effective against Gram-positive bacteria | |
Cytotoxicity | Induces apoptosis in cancer cell lines | |
Enzyme inhibition | Modifies active site residues |
Case Studies
- Antimicrobial Efficacy : A study evaluated the antimicrobial properties of this compound against various bacterial strains. Results indicated significant inhibition of growth, particularly against Staphylococcus aureus and Escherichia coli. The minimum inhibitory concentration (MIC) values ranged from 50 to 100 µg/mL, demonstrating its potential as an antimicrobial agent.
- Cytotoxicity in Cancer Research : In vitro studies have shown that this compound can induce cell death in human breast cancer cells (MCF-7). The compound was found to trigger apoptotic pathways, leading to increased expression of pro-apoptotic proteins and decreased levels of anti-apoptotic factors. The IC50 value was determined to be approximately 20 µM, indicating a promising therapeutic window for further development.
- Enzyme Inhibition Studies : Research exploring the mechanism of action revealed that this compound inhibits acetylcholinesterase (AChE), an enzyme critical for neurotransmission. Kinetic studies showed that BCA acts as a non-competitive inhibitor, providing insights into its potential neuropharmacological applications.
Q & A
Q. What are the standard synthetic routes for benzyl chloroacetate, and how do reaction conditions influence yield?
Basic Research Question
this compound is synthesized via esterification of chloroacetic acid with benzyl alcohol, typically using acid catalysts (e.g., sulfuric acid) under reflux. Key variables include temperature (80–120°C), catalyst concentration, and stoichiometric ratios. For example, excess benzyl alcohol may improve esterification efficiency. The compound’s CAS (140-18-1) and molecular formula (C₉H₉ClO₂) confirm its structural identity . Advanced protocols employ palladium-catalyzed α-arylation with potassium aryltrifluoroborates, where base selection (e.g., K₃PO₄ vs. Cs₂CO₃) significantly impacts conversion rates (Table 1, ).
Q. How can researchers characterize the purity and structural integrity of this compound?
Intermediate Research Question
Methodological Answer:
- Nuclear Magnetic Resonance (NMR): ¹H NMR (CDCl₃) shows characteristic peaks: δ 7.35–7.25 (m, aromatic H), δ 5.25 (s, CH₂O), δ 4.10 (s, ClCH₂COO). ¹³C NMR confirms ester carbonyl (δ 168–170 ppm) .
- Gas Chromatography-Mass Spectrometry (GC-MS): Retention times and fragmentation patterns (e.g., m/z 184 for [M]⁺) validate purity. A study reported 98% purity via GC-MS using a DB-5 column .
- Elemental Analysis: Matches theoretical C (58.55%), H (4.91%), Cl (19.21%) .
Q. What are the challenges in optimizing solvent systems for this compound in catalytic reactions?
Advanced Research Question
Methodological Answer:
Solvent polarity and H-bonding capacity critically influence reaction kinetics. For example, binary mixtures (e.g., ethyl chloroacetate + benzyl alcohol) exhibit non-ideal viscosity and density behavior, requiring empirical optimization (e.g., using the Jouyban-Acree model) . In Pd-catalyzed α-arylation, polar aprotic solvents (e.g., DMF) enhance substrate solubility but may deactivate catalysts. Researchers should conduct systematic solvent screening (e.g., via DoE) to balance reactivity and stability .
Q. How do base additives affect the efficiency of palladium-catalyzed reactions involving this compound?
Advanced Research Question
Methodological Answer:
Base choice governs deprotonation and catalyst turnover. In α-arylation with potassium 2-methoxyphenyltrifluoroborate (Table 1, ):
Base | Conversion (%) |
---|---|
K₃PO₄ | 92 |
Cs₂CO₃ | 85 |
NaOAc | 68 |
Strong bases (K₃PO₄) favor higher conversions but may promote side reactions. Kinetic studies under inert atmospheres (N₂/Ar) are recommended to mitigate oxidation. |
Q. What safety protocols are essential for handling this compound in laboratory settings?
Intermediate Research Question
Methodological Answer:
- Personal Protective Equipment (PPE): Gloves (nitrile), goggles, and lab coats to avoid dermal/ocular exposure .
- Ventilation: Use fume hoods to prevent inhalation of vapors/aerosols .
- Spill Management: Absorb with inert materials (e.g., vermiculite) and dispose as hazardous waste .
- Storage: In sealed containers away from ignition sources, at temperatures <25°C .
Q. How can contradictions in reported reaction yields for this compound be resolved?
Advanced Research Question
Methodological Answer:
Discrepancies often arise from:
- Impurity Profiles: Variations in starting material purity (e.g., benzyl alcohol grade). Validate via GC-MS .
- Catalyst Loading: Pd(PPh₃)₄ vs. Pd(OAc)₂ may alter turnover. Replicate conditions with controlled catalyst batches.
- Analytical Calibration: Standardize GC/MS integration methods to reduce quantification errors .
Q. What future research directions address the limitations of this compound in organic synthesis?
Advanced Research Question
Methodological Answer:
- Green Chemistry: Develop solvent-free esterification or biocatalytic routes using lipases .
- Purification Innovation: Explore column chromatography with gradient elution (hexane/EtOAc) to separate by-products .
- Toxicity Studies: Assess ecotoxicological impacts (e.g., Daphnia magna assays) to guide waste management .
Properties
IUPAC Name |
benzyl 2-chloroacetate | |
---|---|---|
Source | PubChem | |
URL | https://pubchem.ncbi.nlm.nih.gov | |
Description | Data deposited in or computed by PubChem | |
InChI |
InChI=1S/C9H9ClO2/c10-6-9(11)12-7-8-4-2-1-3-5-8/h1-5H,6-7H2 | |
Source | PubChem | |
URL | https://pubchem.ncbi.nlm.nih.gov | |
Description | Data deposited in or computed by PubChem | |
InChI Key |
SOGXBRHOWDEKQB-UHFFFAOYSA-N | |
Source | PubChem | |
URL | https://pubchem.ncbi.nlm.nih.gov | |
Description | Data deposited in or computed by PubChem | |
Canonical SMILES |
C1=CC=C(C=C1)COC(=O)CCl | |
Source | PubChem | |
URL | https://pubchem.ncbi.nlm.nih.gov | |
Description | Data deposited in or computed by PubChem | |
Molecular Formula |
C9H9ClO2 | |
Source | PubChem | |
URL | https://pubchem.ncbi.nlm.nih.gov | |
Description | Data deposited in or computed by PubChem | |
DSSTOX Substance ID |
DTXSID8059689 | |
Record name | Benzyl 2-chloroacetate | |
Source | EPA DSSTox | |
URL | https://comptox.epa.gov/dashboard/DTXSID8059689 | |
Description | DSSTox provides a high quality public chemistry resource for supporting improved predictive toxicology. | |
Molecular Weight |
184.62 g/mol | |
Source | PubChem | |
URL | https://pubchem.ncbi.nlm.nih.gov | |
Description | Data deposited in or computed by PubChem | |
CAS No. |
140-18-1 | |
Record name | Phenylmethyl 2-chloroacetate | |
Source | CAS Common Chemistry | |
URL | https://commonchemistry.cas.org/detail?cas_rn=140-18-1 | |
Description | CAS Common Chemistry is an open community resource for accessing chemical information. Nearly 500,000 chemical substances from CAS REGISTRY cover areas of community interest, including common and frequently regulated chemicals, and those relevant to high school and undergraduate chemistry classes. This chemical information, curated by our expert scientists, is provided in alignment with our mission as a division of the American Chemical Society. | |
Explanation | The data from CAS Common Chemistry is provided under a CC-BY-NC 4.0 license, unless otherwise stated. | |
Record name | Benzyl chloroacetate | |
Source | ChemIDplus | |
URL | https://pubchem.ncbi.nlm.nih.gov/substance/?source=chemidplus&sourceid=0000140181 | |
Description | ChemIDplus is a free, web search system that provides access to the structure and nomenclature authority files used for the identification of chemical substances cited in National Library of Medicine (NLM) databases, including the TOXNET system. | |
Record name | Benzyl chloroacetate | |
Source | DTP/NCI | |
URL | https://dtp.cancer.gov/dtpstandard/servlet/dwindex?searchtype=NSC&outputformat=html&searchlist=8061 | |
Description | The NCI Development Therapeutics Program (DTP) provides services and resources to the academic and private-sector research communities worldwide to facilitate the discovery and development of new cancer therapeutic agents. | |
Explanation | Unless otherwise indicated, all text within NCI products is free of copyright and may be reused without our permission. Credit the National Cancer Institute as the source. | |
Record name | Acetic acid, 2-chloro-, phenylmethyl ester | |
Source | EPA Chemicals under the TSCA | |
URL | https://www.epa.gov/chemicals-under-tsca | |
Description | EPA Chemicals under the Toxic Substances Control Act (TSCA) collection contains information on chemicals and their regulations under TSCA, including non-confidential content from the TSCA Chemical Substance Inventory and Chemical Data Reporting. | |
Record name | Benzyl 2-chloroacetate | |
Source | EPA DSSTox | |
URL | https://comptox.epa.gov/dashboard/DTXSID8059689 | |
Description | DSSTox provides a high quality public chemistry resource for supporting improved predictive toxicology. | |
Record name | Phenylmethyl chloroacetate | |
Source | European Chemicals Agency (ECHA) | |
URL | https://echa.europa.eu/substance-information/-/substanceinfo/100.004.910 | |
Description | The European Chemicals Agency (ECHA) is an agency of the European Union which is the driving force among regulatory authorities in implementing the EU's groundbreaking chemicals legislation for the benefit of human health and the environment as well as for innovation and competitiveness. | |
Explanation | Use of the information, documents and data from the ECHA website is subject to the terms and conditions of this Legal Notice, and subject to other binding limitations provided for under applicable law, the information, documents and data made available on the ECHA website may be reproduced, distributed and/or used, totally or in part, for non-commercial purposes provided that ECHA is acknowledged as the source: "Source: European Chemicals Agency, http://echa.europa.eu/". Such acknowledgement must be included in each copy of the material. ECHA permits and encourages organisations and individuals to create links to the ECHA website under the following cumulative conditions: Links can only be made to webpages that provide a link to the Legal Notice page. | |
Record name | Benzyl chloroacetate | |
Source | FDA Global Substance Registration System (GSRS) | |
URL | https://gsrs.ncats.nih.gov/ginas/app/beta/substances/B52EJ32AB5 | |
Description | The FDA Global Substance Registration System (GSRS) enables the efficient and accurate exchange of information on what substances are in regulated products. Instead of relying on names, which vary across regulatory domains, countries, and regions, the GSRS knowledge base makes it possible for substances to be defined by standardized, scientific descriptions. | |
Explanation | Unless otherwise noted, the contents of the FDA website (www.fda.gov), both text and graphics, are not copyrighted. They are in the public domain and may be republished, reprinted and otherwise used freely by anyone without the need to obtain permission from FDA. Credit to the U.S. Food and Drug Administration as the source is appreciated but not required. | |
Synthesis routes and methods
Procedure details
Retrosynthesis Analysis
AI-Powered Synthesis Planning: Our tool employs the Template_relevance Pistachio, Template_relevance Bkms_metabolic, Template_relevance Pistachio_ringbreaker, Template_relevance Reaxys, Template_relevance Reaxys_biocatalysis model, leveraging a vast database of chemical reactions to predict feasible synthetic routes.
One-Step Synthesis Focus: Specifically designed for one-step synthesis, it provides concise and direct routes for your target compounds, streamlining the synthesis process.
Accurate Predictions: Utilizing the extensive PISTACHIO, BKMS_METABOLIC, PISTACHIO_RINGBREAKER, REAXYS, REAXYS_BIOCATALYSIS database, our tool offers high-accuracy predictions, reflecting the latest in chemical research and data.
Strategy Settings
Precursor scoring | Relevance Heuristic |
---|---|
Min. plausibility | 0.01 |
Model | Template_relevance |
Template Set | Pistachio/Bkms_metabolic/Pistachio_ringbreaker/Reaxys/Reaxys_biocatalysis |
Top-N result to add to graph | 6 |
Feasible Synthetic Routes
Disclaimer and Information on In-Vitro Research Products
Please be aware that all articles and product information presented on BenchChem are intended solely for informational purposes. The products available for purchase on BenchChem are specifically designed for in-vitro studies, which are conducted outside of living organisms. In-vitro studies, derived from the Latin term "in glass," involve experiments performed in controlled laboratory settings using cells or tissues. It is important to note that these products are not categorized as medicines or drugs, and they have not received approval from the FDA for the prevention, treatment, or cure of any medical condition, ailment, or disease. We must emphasize that any form of bodily introduction of these products into humans or animals is strictly prohibited by law. It is essential to adhere to these guidelines to ensure compliance with legal and ethical standards in research and experimentation.