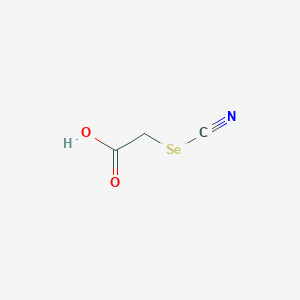
Selenocyanatoacetic acid
Overview
Description
Scientific Research Applications
Synthetic Auxin Production : Selenium compounds, such as 2,4-dichlorophenylselenoacetic acid, have been synthesized and tested as auxins (plant growth regulators) in bioassays, demonstrating strong synthetic auxin properties (Tadino et al., 2003).
Cancer Chemoprevention : Organoselenium compounds, including selenocyanates, have shown potential in cancer prevention and treatment due to their antioxidant, prooxidant, and redox-modulating properties (Álvarez-Pérez et al., 2018).
Biomedical Applications : Selenium nanoparticles, less toxic than inorganic and organic selenium compounds, are being explored for their therapeutic potential in cancer therapies (Ferro et al., 2021).
Antioxidant Activity : Selenium, including organoselenium compounds, plays a crucial role as an antioxidant in human health, protecting against oxidative stress initiated by reactive oxygen and nitrogen species (Tinggi, 2008).
Immune System Enhancement : Selenium compounds have been used to enhance immune responses, as seen in studies involving selenium Hericium erinaceus polysaccharide nanoparticles (Luo et al., 2019).
Agricultural Biofortification : Enhancing soil selenium availability has been researched as a strategy to promote selenium uptake in plants, particularly in selenium-enriched rice cultivars (Mu et al., 2019).
Selenoprotein Research : Understanding the molecular pathways and physiological roles of selenoproteins, which contain selenium as selenocysteine, is crucial in elucidating their roles in human health (Labunskyy et al., 2014).
properties
IUPAC Name |
2-selenocyanatoacetic acid | |
---|---|---|
Source | PubChem | |
URL | https://pubchem.ncbi.nlm.nih.gov | |
Description | Data deposited in or computed by PubChem | |
InChI |
InChI=1S/C3H3NO2Se/c4-2-7-1-3(5)6/h1H2,(H,5,6) | |
Source | PubChem | |
URL | https://pubchem.ncbi.nlm.nih.gov | |
Description | Data deposited in or computed by PubChem | |
InChI Key |
VSZTUOPTOVFUEE-UHFFFAOYSA-N | |
Source | PubChem | |
URL | https://pubchem.ncbi.nlm.nih.gov | |
Description | Data deposited in or computed by PubChem | |
Canonical SMILES |
C(C(=O)O)[Se]C#N | |
Source | PubChem | |
URL | https://pubchem.ncbi.nlm.nih.gov | |
Description | Data deposited in or computed by PubChem | |
Molecular Formula |
C3H3NO2Se | |
Source | PubChem | |
URL | https://pubchem.ncbi.nlm.nih.gov | |
Description | Data deposited in or computed by PubChem | |
DSSTOX Substance ID |
DTXSID40275477 | |
Record name | selenocyanatoacetic acid | |
Source | EPA DSSTox | |
URL | https://comptox.epa.gov/dashboard/DTXSID40275477 | |
Description | DSSTox provides a high quality public chemistry resource for supporting improved predictive toxicology. | |
Molecular Weight |
164.03 g/mol | |
Source | PubChem | |
URL | https://pubchem.ncbi.nlm.nih.gov | |
Description | Data deposited in or computed by PubChem | |
Product Name |
Selenocyanatoacetic acid | |
CAS RN |
19188-17-1 | |
Record name | selenocyanatoacetic acid | |
Source | EPA DSSTox | |
URL | https://comptox.epa.gov/dashboard/DTXSID40275477 | |
Description | DSSTox provides a high quality public chemistry resource for supporting improved predictive toxicology. | |
Retrosynthesis Analysis
AI-Powered Synthesis Planning: Our tool employs the Template_relevance Pistachio, Template_relevance Bkms_metabolic, Template_relevance Pistachio_ringbreaker, Template_relevance Reaxys, Template_relevance Reaxys_biocatalysis model, leveraging a vast database of chemical reactions to predict feasible synthetic routes.
One-Step Synthesis Focus: Specifically designed for one-step synthesis, it provides concise and direct routes for your target compounds, streamlining the synthesis process.
Accurate Predictions: Utilizing the extensive PISTACHIO, BKMS_METABOLIC, PISTACHIO_RINGBREAKER, REAXYS, REAXYS_BIOCATALYSIS database, our tool offers high-accuracy predictions, reflecting the latest in chemical research and data.
Strategy Settings
Precursor scoring | Relevance Heuristic |
---|---|
Min. plausibility | 0.01 |
Model | Template_relevance |
Template Set | Pistachio/Bkms_metabolic/Pistachio_ringbreaker/Reaxys/Reaxys_biocatalysis |
Top-N result to add to graph | 6 |
Feasible Synthetic Routes
Disclaimer and Information on In-Vitro Research Products
Please be aware that all articles and product information presented on BenchChem are intended solely for informational purposes. The products available for purchase on BenchChem are specifically designed for in-vitro studies, which are conducted outside of living organisms. In-vitro studies, derived from the Latin term "in glass," involve experiments performed in controlled laboratory settings using cells or tissues. It is important to note that these products are not categorized as medicines or drugs, and they have not received approval from the FDA for the prevention, treatment, or cure of any medical condition, ailment, or disease. We must emphasize that any form of bodily introduction of these products into humans or animals is strictly prohibited by law. It is essential to adhere to these guidelines to ensure compliance with legal and ethical standards in research and experimentation.