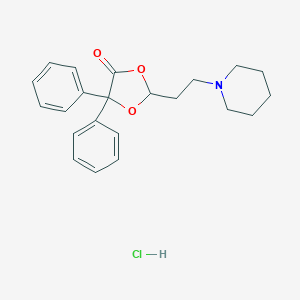
Pipoxolan hydrochloride
Overview
Description
Pipoxolan hydrochloride is a pharmacological compound known for its antispasmodic properties. It is primarily used to relieve smooth muscle spasms in various parts of the body, including the gastrointestinal tract, gallbladder, urogenital area, and bronchial passages . The compound has also shown potential in treating cerebrovascular diseases and certain types of cancer .
Preparation Methods
Synthetic Routes and Reaction Conditions
The synthesis of pipoxolan hydrochloride involves the reaction of 5,5-diphenyl-2-(2-piperidin-1-ylethyl)-1,3-dioxolan-4-one with hydrochloric acid. The reaction typically occurs under controlled conditions to ensure the formation of the hydrochloride salt .
Industrial Production Methods
Industrial production of this compound follows a similar synthetic route but on a larger scale. The process involves the use of high-purity reagents and stringent quality control measures to ensure the consistency and purity of the final product .
Chemical Reactions Analysis
Types of Reactions
Pipoxolan hydrochloride undergoes various chemical reactions, including:
Oxidation: The compound can be oxidized under specific conditions to form different oxidation products.
Reduction: Reduction reactions can be performed to modify the functional groups within the molecule.
Substitution: Substitution reactions, particularly nucleophilic substitutions, can occur at specific sites on the molecule.
Common Reagents and Conditions
Oxidation: Hydrogen peroxide or other oxidizing agents.
Reduction: Sodium borohydride or other reducing agents.
Substitution: Nucleophiles such as amines or thiols.
Major Products Formed
The major products formed from these reactions depend on the specific conditions and reagents used. For example, oxidation may yield hydroxylated derivatives, while reduction can produce deoxygenated compounds .
Scientific Research Applications
Pipoxolan hydrochloride has a wide range of scientific research applications:
Chemistry: Used as a model compound to study antispasmodic agents and their mechanisms.
Industry: Utilized in the development of new pharmaceuticals and therapeutic agents.
Mechanism of Action
Pipoxolan hydrochloride primarily functions as a smooth muscle relaxant. It achieves this by inhibiting calcium channels within muscle cells, reducing the influx of calcium ions, and thereby decreasing muscle contraction . Additionally, the compound enhances cyclic adenosine monophosphate levels by inhibiting phosphodiesterase enzymes, leading to further muscle relaxation . This dual mechanism ensures a robust and sustained relaxation effect on smooth muscles.
Comparison with Similar Compounds
Similar Compounds
Dicyclomine hydrochloride: Another antispasmodic agent used to treat gastrointestinal spasms.
Hyoscine butylbromide: Used for its antispasmodic properties in treating abdominal pain and cramps.
Papaverine hydrochloride: A smooth muscle relaxant used to treat vasospasms and other conditions.
Uniqueness
Pipoxolan hydrochloride is unique due to its dual mechanism of action, involving both calcium channel inhibition and enhancement of cyclic adenosine monophosphate levels. This makes it particularly effective in providing sustained muscle relaxation compared to other antispasmodic agents .
Properties
IUPAC Name |
5,5-diphenyl-2-(2-piperidin-1-ylethyl)-1,3-dioxolan-4-one;hydrochloride | |
---|---|---|
Source | PubChem | |
URL | https://pubchem.ncbi.nlm.nih.gov | |
Description | Data deposited in or computed by PubChem | |
InChI |
InChI=1S/C22H25NO3.ClH/c24-21-22(18-10-4-1-5-11-18,19-12-6-2-7-13-19)26-20(25-21)14-17-23-15-8-3-9-16-23;/h1-2,4-7,10-13,20H,3,8-9,14-17H2;1H | |
Source | PubChem | |
URL | https://pubchem.ncbi.nlm.nih.gov | |
Description | Data deposited in or computed by PubChem | |
InChI Key |
JXNQXJVDHXGEPU-UHFFFAOYSA-N | |
Source | PubChem | |
URL | https://pubchem.ncbi.nlm.nih.gov | |
Description | Data deposited in or computed by PubChem | |
Canonical SMILES |
C1CCN(CC1)CCC2OC(=O)C(O2)(C3=CC=CC=C3)C4=CC=CC=C4.Cl | |
Source | PubChem | |
URL | https://pubchem.ncbi.nlm.nih.gov | |
Description | Data deposited in or computed by PubChem | |
Molecular Formula |
C22H26ClNO3 | |
Source | PubChem | |
URL | https://pubchem.ncbi.nlm.nih.gov | |
Description | Data deposited in or computed by PubChem | |
Related CAS |
23744-24-3 (Parent) | |
Record name | Pipoxolan hydrochloride [USAN] | |
Source | ChemIDplus | |
URL | https://pubchem.ncbi.nlm.nih.gov/substance/?source=chemidplus&sourceid=0018174588 | |
Description | ChemIDplus is a free, web search system that provides access to the structure and nomenclature authority files used for the identification of chemical substances cited in National Library of Medicine (NLM) databases, including the TOXNET system. | |
DSSTOX Substance ID |
DTXSID5057672 | |
Record name | Pipoxolan hydrochloride | |
Source | EPA DSSTox | |
URL | https://comptox.epa.gov/dashboard/DTXSID5057672 | |
Description | DSSTox provides a high quality public chemistry resource for supporting improved predictive toxicology. | |
Molecular Weight |
387.9 g/mol | |
Source | PubChem | |
URL | https://pubchem.ncbi.nlm.nih.gov | |
Description | Data deposited in or computed by PubChem | |
CAS No. |
18174-58-8 | |
Record name | Pipoxolan hydrochloride [USAN] | |
Source | ChemIDplus | |
URL | https://pubchem.ncbi.nlm.nih.gov/substance/?source=chemidplus&sourceid=0018174588 | |
Description | ChemIDplus is a free, web search system that provides access to the structure and nomenclature authority files used for the identification of chemical substances cited in National Library of Medicine (NLM) databases, including the TOXNET system. | |
Record name | Pipoxolan hydrochloride | |
Source | EPA DSSTox | |
URL | https://comptox.epa.gov/dashboard/DTXSID5057672 | |
Description | DSSTox provides a high quality public chemistry resource for supporting improved predictive toxicology. | |
Record name | PIPOXOLAN HYDROCHLORIDE | |
Source | FDA Global Substance Registration System (GSRS) | |
URL | https://gsrs.ncats.nih.gov/ginas/app/beta/substances/VIA6WM647S | |
Description | The FDA Global Substance Registration System (GSRS) enables the efficient and accurate exchange of information on what substances are in regulated products. Instead of relying on names, which vary across regulatory domains, countries, and regions, the GSRS knowledge base makes it possible for substances to be defined by standardized, scientific descriptions. | |
Explanation | Unless otherwise noted, the contents of the FDA website (www.fda.gov), both text and graphics, are not copyrighted. They are in the public domain and may be republished, reprinted and otherwise used freely by anyone without the need to obtain permission from FDA. Credit to the U.S. Food and Drug Administration as the source is appreciated but not required. | |
Retrosynthesis Analysis
AI-Powered Synthesis Planning: Our tool employs the Template_relevance Pistachio, Template_relevance Bkms_metabolic, Template_relevance Pistachio_ringbreaker, Template_relevance Reaxys, Template_relevance Reaxys_biocatalysis model, leveraging a vast database of chemical reactions to predict feasible synthetic routes.
One-Step Synthesis Focus: Specifically designed for one-step synthesis, it provides concise and direct routes for your target compounds, streamlining the synthesis process.
Accurate Predictions: Utilizing the extensive PISTACHIO, BKMS_METABOLIC, PISTACHIO_RINGBREAKER, REAXYS, REAXYS_BIOCATALYSIS database, our tool offers high-accuracy predictions, reflecting the latest in chemical research and data.
Strategy Settings
Precursor scoring | Relevance Heuristic |
---|---|
Min. plausibility | 0.01 |
Model | Template_relevance |
Template Set | Pistachio/Bkms_metabolic/Pistachio_ringbreaker/Reaxys/Reaxys_biocatalysis |
Top-N result to add to graph | 6 |
Feasible Synthetic Routes
Disclaimer and Information on In-Vitro Research Products
Please be aware that all articles and product information presented on BenchChem are intended solely for informational purposes. The products available for purchase on BenchChem are specifically designed for in-vitro studies, which are conducted outside of living organisms. In-vitro studies, derived from the Latin term "in glass," involve experiments performed in controlled laboratory settings using cells or tissues. It is important to note that these products are not categorized as medicines or drugs, and they have not received approval from the FDA for the prevention, treatment, or cure of any medical condition, ailment, or disease. We must emphasize that any form of bodily introduction of these products into humans or animals is strictly prohibited by law. It is essential to adhere to these guidelines to ensure compliance with legal and ethical standards in research and experimentation.