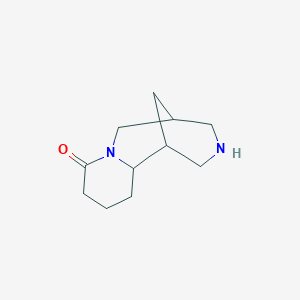
Tetrahydrocytisine
Overview
Description
Tetrahydrocytisine is a quinolizidine alkaloid that is structurally related to cytisine. It is known for its ability to selectively affect nicotinic cholinergic receptors, making it a compound of interest in pharmacology and synthetic chemistry .
Preparation Methods
Tetrahydrocytisine can be synthesized from quinolizidine-2,6-dione through a series of steps. One common synthetic route involves a double Mannich condensation reaction . The key intermediate, quinolizidine-2,6-dione, can be prepared using a biomimetic α-acylimmonium cyclization method . This involves the alkylation of glutarimide with 3-butyn-1-ol using a Mitsunobu protocol, followed by reduction with L-Selectride and treatment with formic acid . The resulting product undergoes further reactions to yield this compound .
Chemical Reactions Analysis
Tetrahydrocytisine undergoes various chemical reactions, including oxidation, reduction, and substitution reactions. For example, it can be arylated by 2-fluoro-5-nitrobenzaldehyde and subsequently condensed with 1,3-dimethylbarbituric acid . This reaction produces spirocyclic products with different skeletons, such as the lupanine skeleton and its regioisomer . The reactions are often stereoselective, and the structures of the products can be confirmed using nuclear magnetic resonance and X-ray crystal structure analyses .
Scientific Research Applications
Tetrahydrocytisine has several scientific research applications. It has been studied for its potential use in developing new drugs for smoking cessation due to its ability to affect nicotinic cholinergic receptors . Additionally, it has shown antifungal activity against Fusarium oxysporum, making it a candidate for agricultural applications . The compound’s derivatives have also been explored for their potential in synthesizing new heterocyclic quinolizidine systems .
Mechanism of Action
The mechanism of action of tetrahydrocytisine involves its interaction with nicotinic cholinergic receptors. By binding to these receptors, it can modulate their activity, which is crucial for its pharmacological effects . The compound’s ability to selectively target these receptors makes it a valuable tool in studying the cholinergic system and developing related therapies .
Comparison with Similar Compounds
Tetrahydrocytisine is similar to other quinolizidine alkaloids such as cytisine, lupanine, and sparteine . its unique ability to selectively affect nicotinic cholinergic receptors sets it apart from these compounds . This selectivity makes this compound particularly valuable in pharmacological research and drug development .
Properties
IUPAC Name |
7,11-diazatricyclo[7.3.1.02,7]tridecan-6-one | |
---|---|---|
Source | PubChem | |
URL | https://pubchem.ncbi.nlm.nih.gov | |
Description | Data deposited in or computed by PubChem | |
InChI |
InChI=1S/C11H18N2O/c14-11-3-1-2-10-9-4-8(5-12-6-9)7-13(10)11/h8-10,12H,1-7H2 | |
Source | PubChem | |
URL | https://pubchem.ncbi.nlm.nih.gov | |
Description | Data deposited in or computed by PubChem | |
InChI Key |
KWVYCGMBGRYVQH-UHFFFAOYSA-N | |
Source | PubChem | |
URL | https://pubchem.ncbi.nlm.nih.gov | |
Description | Data deposited in or computed by PubChem | |
Canonical SMILES |
C1CC2C3CC(CNC3)CN2C(=O)C1 | |
Source | PubChem | |
URL | https://pubchem.ncbi.nlm.nih.gov | |
Description | Data deposited in or computed by PubChem | |
Molecular Formula |
C11H18N2O | |
Source | PubChem | |
URL | https://pubchem.ncbi.nlm.nih.gov | |
Description | Data deposited in or computed by PubChem | |
Molecular Weight |
194.27 g/mol | |
Source | PubChem | |
URL | https://pubchem.ncbi.nlm.nih.gov | |
Description | Data deposited in or computed by PubChem | |
Retrosynthesis Analysis
AI-Powered Synthesis Planning: Our tool employs the Template_relevance Pistachio, Template_relevance Bkms_metabolic, Template_relevance Pistachio_ringbreaker, Template_relevance Reaxys, Template_relevance Reaxys_biocatalysis model, leveraging a vast database of chemical reactions to predict feasible synthetic routes.
One-Step Synthesis Focus: Specifically designed for one-step synthesis, it provides concise and direct routes for your target compounds, streamlining the synthesis process.
Accurate Predictions: Utilizing the extensive PISTACHIO, BKMS_METABOLIC, PISTACHIO_RINGBREAKER, REAXYS, REAXYS_BIOCATALYSIS database, our tool offers high-accuracy predictions, reflecting the latest in chemical research and data.
Strategy Settings
Precursor scoring | Relevance Heuristic |
---|---|
Min. plausibility | 0.01 |
Model | Template_relevance |
Template Set | Pistachio/Bkms_metabolic/Pistachio_ringbreaker/Reaxys/Reaxys_biocatalysis |
Top-N result to add to graph | 6 |
Feasible Synthetic Routes
Q1: What is the background and significance of tetrahydrocytisine?
A1: this compound (THC) is a quinolizidine alkaloid, a class of naturally occurring nitrogen-containing compounds found in various plants and animals. These alkaloids exhibit diverse biological activities, including cytotoxic, antiviral, antimicrobial, and insecticidal properties []. THC itself has garnered interest for its potential therapeutic applications and serves as a valuable building block in organic synthesis. [, ].
Q2: Can you describe a synthetic route for this compound?
A2: A concise synthesis of this compound (5) starts with quinolizidine-2,6-dione as the precursor. This route proceeds through three steps and achieves a reasonable overall yield [].
Q3: What are the potential applications of this compound derivatives?
A3: Researchers have explored the use of this compound as a scaffold for novel diazaadamantanes []. These compounds hold promise for various applications, though specific details might be limited at this stage of research.
Q4: Has the fragmentation pattern of this compound been studied?
A4: Yes, mass spectrometry studies have investigated the fragmentation patterns of this compound and related quinolizidine alkaloids, offering insights into their structural characteristics [].
Q5: Are there any known biological activities associated with this compound?
A5: While specific details on this compound's biological activities might be limited in the provided abstracts, the broader class of quinolizidine alkaloids, to which it belongs, exhibits a wide range of activities. These include antifungal effects, as demonstrated against the plant pathogen Fusarium oxysporum [].
Q6: Are there studies on the chemical modification of this compound?
A6: Researchers have investigated the acid hydrolysis of this compound and its N-derivatives, providing insights into its reactivity and potential for structural modifications []. Further exploration involves stereocontrolled T-reactions of (1R,5S,12S)-tetrahydrocytisine derivatives, enabling the synthesis of new analogs with potentially modified biological properties [, ].
Disclaimer and Information on In-Vitro Research Products
Please be aware that all articles and product information presented on BenchChem are intended solely for informational purposes. The products available for purchase on BenchChem are specifically designed for in-vitro studies, which are conducted outside of living organisms. In-vitro studies, derived from the Latin term "in glass," involve experiments performed in controlled laboratory settings using cells or tissues. It is important to note that these products are not categorized as medicines or drugs, and they have not received approval from the FDA for the prevention, treatment, or cure of any medical condition, ailment, or disease. We must emphasize that any form of bodily introduction of these products into humans or animals is strictly prohibited by law. It is essential to adhere to these guidelines to ensure compliance with legal and ethical standards in research and experimentation.