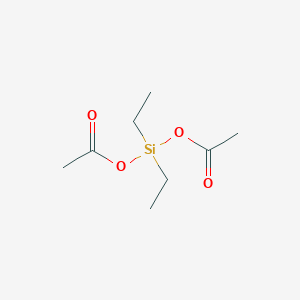
Diacetoxydiethylsilane
Overview
Description
Diacetoxydiethylsilane is an organosilicon compound with the molecular formula C8H16O4Si. It is characterized by the presence of two acetoxy groups attached to a silicon atom, which is also bonded to two ethyl groups. This compound is a colorless to light yellow liquid with a distinct odor of acetic acid. This compound is primarily used in various chemical synthesis processes and has applications in the production of silicone-based materials.
Preparation Methods
Synthetic Routes and Reaction Conditions: Diacetoxydiethylsilane can be synthesized through the reaction of diethylsilane with acetic anhydride. The reaction typically occurs under anhydrous conditions to prevent hydrolysis of the acetoxy groups. The general reaction is as follows:
(C2H5)2SiH2 + 2 (CH3CO)2O → (C2H5)2Si(OCOCH3)2 + 2 CH3COOH
The reaction is carried out at elevated temperatures, usually around 60-80°C, and requires a catalyst such as pyridine to facilitate the acetylation process.
Industrial Production Methods: In industrial settings, this compound is produced in large quantities using continuous flow reactors. The process involves the controlled addition of diethylsilane and acetic anhydride into the reactor, where the reaction takes place under optimized temperature and pressure conditions. The product is then purified through distillation to remove any unreacted starting materials and by-products.
Types of Reactions:
Hydrolysis: this compound undergoes hydrolysis in the presence of water, leading to the formation of diethylsilanediol and acetic acid.
(C2H5)2Si(OCOCH3)2 + 2 H2O → (C2H5)2Si(OH)2 + 2 CH3COOH
Condensation: The silanol groups formed from hydrolysis can further condense to form siloxane bonds, leading to the formation of polymeric structures.
2 (C2H5)2Si(OH)2 → (C2H5)2Si-O-Si(C2H5)2 + 2 H2O
Substitution: this compound can undergo substitution reactions with nucleophiles such as alcohols or amines, replacing the acetoxy groups with alkoxy or amino groups.
Common Reagents and Conditions:
Hydrolysis: Water or aqueous solutions, typically at room temperature.
Condensation: Catalysts such as acids or bases, with controlled temperature and humidity.
Substitution: Alcohols or amines, often in the presence of a base such as triethylamine.
Major Products Formed:
Hydrolysis: Diethylsilanediol and acetic acid.
Condensation: Polymeric siloxanes.
Substitution: Alkoxysilanes or aminosilanes, depending on the nucleophile used.
Scientific Research Applications
Diacetoxydiethylsilane has a wide range of applications in scientific research and industry:
Chemistry: Used as a precursor for the synthesis of various organosilicon compounds and silicone-based materials.
Biology: Employed in the modification of surfaces for biological assays and the development of biosensors.
Medicine: Investigated for its potential use in drug delivery systems and as a component in medical devices.
Industry: Utilized in the production of silicone sealants, adhesives, and coatings due to its ability to form strong siloxane bonds.
Mechanism of Action
The mechanism of action of diacetoxydiethylsilane primarily involves its ability to undergo hydrolysis and condensation reactions. Upon exposure to moisture, the acetoxy groups are hydrolyzed to form silanol groups, which can then condense to form siloxane bonds. This property is crucial for its use in the production of silicone-based materials, as it allows for the formation of durable and flexible polymeric structures.
Comparison with Similar Compounds
Diacetoxydimethylsilane: Similar in structure but with methyl groups instead of ethyl groups.
Diethoxydimethylsilane: Contains ethoxy groups instead of acetoxy groups.
Dimethyldiacetoxysilane: Similar to diacetoxydiethylsilane but with methyl groups.
Uniqueness: this compound is unique due to the presence of ethyl groups, which can influence the reactivity and physical properties of the compound. The ethyl groups provide a balance between hydrophobicity and reactivity, making this compound suitable for specific applications where other similar compounds may not perform as effectively.
Properties
IUPAC Name |
[acetyloxy(diethyl)silyl] acetate | |
---|---|---|
Source | PubChem | |
URL | https://pubchem.ncbi.nlm.nih.gov | |
Description | Data deposited in or computed by PubChem | |
InChI |
InChI=1S/C8H16O4Si/c1-5-13(6-2,11-7(3)9)12-8(4)10/h5-6H2,1-4H3 | |
Source | PubChem | |
URL | https://pubchem.ncbi.nlm.nih.gov | |
Description | Data deposited in or computed by PubChem | |
InChI Key |
JQNJIBYLKBOSCM-UHFFFAOYSA-N | |
Source | PubChem | |
URL | https://pubchem.ncbi.nlm.nih.gov | |
Description | Data deposited in or computed by PubChem | |
Canonical SMILES |
CC[Si](CC)(OC(=O)C)OC(=O)C | |
Source | PubChem | |
URL | https://pubchem.ncbi.nlm.nih.gov | |
Description | Data deposited in or computed by PubChem | |
Molecular Formula |
C8H16O4Si | |
Source | PubChem | |
URL | https://pubchem.ncbi.nlm.nih.gov | |
Description | Data deposited in or computed by PubChem | |
DSSTOX Substance ID |
DTXSID30171318 | |
Record name | Diacetoxydiethylsilane | |
Source | EPA DSSTox | |
URL | https://comptox.epa.gov/dashboard/DTXSID30171318 | |
Description | DSSTox provides a high quality public chemistry resource for supporting improved predictive toxicology. | |
Molecular Weight |
204.30 g/mol | |
Source | PubChem | |
URL | https://pubchem.ncbi.nlm.nih.gov | |
Description | Data deposited in or computed by PubChem | |
CAS No. |
18269-81-3 | |
Record name | Silanediol, 1,1-diethyl-, 1,1-diacetate | |
Source | CAS Common Chemistry | |
URL | https://commonchemistry.cas.org/detail?cas_rn=18269-81-3 | |
Description | CAS Common Chemistry is an open community resource for accessing chemical information. Nearly 500,000 chemical substances from CAS REGISTRY cover areas of community interest, including common and frequently regulated chemicals, and those relevant to high school and undergraduate chemistry classes. This chemical information, curated by our expert scientists, is provided in alignment with our mission as a division of the American Chemical Society. | |
Explanation | The data from CAS Common Chemistry is provided under a CC-BY-NC 4.0 license, unless otherwise stated. | |
Record name | Diacetoxydiethylsilane | |
Source | ChemIDplus | |
URL | https://pubchem.ncbi.nlm.nih.gov/substance/?source=chemidplus&sourceid=0018269813 | |
Description | ChemIDplus is a free, web search system that provides access to the structure and nomenclature authority files used for the identification of chemical substances cited in National Library of Medicine (NLM) databases, including the TOXNET system. | |
Record name | Diacetoxydiethylsilane | |
Source | EPA DSSTox | |
URL | https://comptox.epa.gov/dashboard/DTXSID30171318 | |
Description | DSSTox provides a high quality public chemistry resource for supporting improved predictive toxicology. | |
Record name | Diacetoxydiethylsilane | |
Source | European Chemicals Agency (ECHA) | |
URL | https://echa.europa.eu/substance-information/-/substanceinfo/100.038.303 | |
Description | The European Chemicals Agency (ECHA) is an agency of the European Union which is the driving force among regulatory authorities in implementing the EU's groundbreaking chemicals legislation for the benefit of human health and the environment as well as for innovation and competitiveness. | |
Explanation | Use of the information, documents and data from the ECHA website is subject to the terms and conditions of this Legal Notice, and subject to other binding limitations provided for under applicable law, the information, documents and data made available on the ECHA website may be reproduced, distributed and/or used, totally or in part, for non-commercial purposes provided that ECHA is acknowledged as the source: "Source: European Chemicals Agency, http://echa.europa.eu/". Such acknowledgement must be included in each copy of the material. ECHA permits and encourages organisations and individuals to create links to the ECHA website under the following cumulative conditions: Links can only be made to webpages that provide a link to the Legal Notice page. | |
Disclaimer and Information on In-Vitro Research Products
Please be aware that all articles and product information presented on BenchChem are intended solely for informational purposes. The products available for purchase on BenchChem are specifically designed for in-vitro studies, which are conducted outside of living organisms. In-vitro studies, derived from the Latin term "in glass," involve experiments performed in controlled laboratory settings using cells or tissues. It is important to note that these products are not categorized as medicines or drugs, and they have not received approval from the FDA for the prevention, treatment, or cure of any medical condition, ailment, or disease. We must emphasize that any form of bodily introduction of these products into humans or animals is strictly prohibited by law. It is essential to adhere to these guidelines to ensure compliance with legal and ethical standards in research and experimentation.