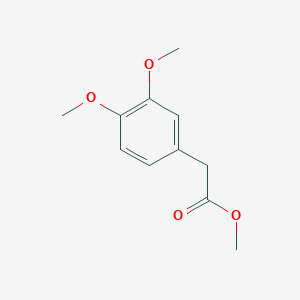
Methyl 2-(3,4-dimethoxyphenyl)acetate
Overview
Description
“Methyl 2-(3,4-dimethoxyphenyl)acetate” is a chemical compound with the CAS Number: 15964-79-1 . It has a molecular weight of 210.23 and its IUPAC name is methyl (3,4-dimethoxyphenyl)acetate .
Molecular Structure Analysis
The molecular formula of “Methyl 2-(3,4-dimethoxyphenyl)acetate” is C11H14O4 . Its InChI code is 1S/C11H14O4/c1-13-9-5-4-8(6-10(9)14-2)7-11(12)15-3/h4-6H,7H2,1-3H3 and its canonical SMILES representation is COC1=C(C=C(C=C1)CC(=O)OC)OC .Physical And Chemical Properties Analysis
“Methyl 2-(3,4-dimethoxyphenyl)acetate” is a light yellow to yellow to brown liquid .Scientific Research Applications
Organic Synthesis Intermediate
“Methyl 2-(3,4-dimethoxyphenyl)acetate” can be used as an important intermediate in organic synthesis . This means it can be used in the production of a variety of other chemical compounds.
Precursor to Cytotoxic Natural Products
A study has developed a methodology for the synthesis of methyl (E)-3-(2,4-dimethoxy-5-nitrophenyl)acrylate, which is a precursor to cytotoxic natural products known as carpatamides A–D . This suggests that “Methyl 2-(3,4-dimethoxyphenyl)acetate” could potentially be used in the synthesis of these cytotoxic compounds.
Labeling Compound in Life Science Research
“Methyl 2-(3,4-dimethoxyphenyl)acetate” can also be used as a labeling compound in life science research . This could involve using the compound to track or measure biological processes.
Research in Palladium-Catalyzed Reactions
The compound has been used in research related to palladium-catalyzed reactions . These types of reactions are important in many areas of chemistry, including the synthesis of pharmaceuticals and fine chemicals.
Safety and Hazards
Mechanism of Action
Target of Action
Methyl 2-(3,4-dimethoxyphenyl)acetate is a chemical compound used in organic synthesis It’s known to be used as an important intermediate in organic synthesis .
Mode of Action
It’s generally used as a building block in the synthesis of other complex molecules . The compound’s interaction with its targets and the resulting changes would depend on the specific synthesis process it’s involved in.
Biochemical Pathways
The biochemical pathways affected by Methyl 2-(3,4-dimethoxyphenyl)acetate would depend on the specific molecules it’s used to synthesize. As an intermediate, it could be involved in various biochemical pathways depending on its application in the synthesis of different target molecules .
Pharmacokinetics
As an intermediate in organic synthesis, its bioavailability would likely depend on the properties of the final synthesized molecule .
Action Environment
The action, efficacy, and stability of Methyl 2-(3,4-dimethoxyphenyl)acetate can be influenced by various environmental factors. These factors could include temperature, pH, and the presence of other chemicals. For instance, it’s recommended to be stored at a temperature between 0-10°C .
properties
IUPAC Name |
methyl 2-(3,4-dimethoxyphenyl)acetate | |
---|---|---|
Source | PubChem | |
URL | https://pubchem.ncbi.nlm.nih.gov | |
Description | Data deposited in or computed by PubChem | |
InChI |
InChI=1S/C11H14O4/c1-13-9-5-4-8(6-10(9)14-2)7-11(12)15-3/h4-6H,7H2,1-3H3 | |
Source | PubChem | |
URL | https://pubchem.ncbi.nlm.nih.gov | |
Description | Data deposited in or computed by PubChem | |
InChI Key |
DILOFCBIBDMHAY-UHFFFAOYSA-N | |
Source | PubChem | |
URL | https://pubchem.ncbi.nlm.nih.gov | |
Description | Data deposited in or computed by PubChem | |
Canonical SMILES |
COC1=C(C=C(C=C1)CC(=O)OC)OC | |
Source | PubChem | |
URL | https://pubchem.ncbi.nlm.nih.gov | |
Description | Data deposited in or computed by PubChem | |
Molecular Formula |
C11H14O4 | |
Source | PubChem | |
URL | https://pubchem.ncbi.nlm.nih.gov | |
Description | Data deposited in or computed by PubChem | |
DSSTOX Substance ID |
DTXSID5065982 | |
Record name | Benzeneacetic acid, 3,4-dimethoxy-, methyl ester | |
Source | EPA DSSTox | |
URL | https://comptox.epa.gov/dashboard/DTXSID5065982 | |
Description | DSSTox provides a high quality public chemistry resource for supporting improved predictive toxicology. | |
Molecular Weight |
210.23 g/mol | |
Source | PubChem | |
URL | https://pubchem.ncbi.nlm.nih.gov | |
Description | Data deposited in or computed by PubChem | |
Product Name |
Methyl 2-(3,4-dimethoxyphenyl)acetate | |
CAS RN |
15964-79-1 | |
Record name | Methyl 2-(3,4-dimethoxyphenyl)acetate | |
Source | CAS Common Chemistry | |
URL | https://commonchemistry.cas.org/detail?cas_rn=15964-79-1 | |
Description | CAS Common Chemistry is an open community resource for accessing chemical information. Nearly 500,000 chemical substances from CAS REGISTRY cover areas of community interest, including common and frequently regulated chemicals, and those relevant to high school and undergraduate chemistry classes. This chemical information, curated by our expert scientists, is provided in alignment with our mission as a division of the American Chemical Society. | |
Explanation | The data from CAS Common Chemistry is provided under a CC-BY-NC 4.0 license, unless otherwise stated. | |
Record name | Benzeneacetic acid, 3,4-dimethoxy-, methyl ester | |
Source | ChemIDplus | |
URL | https://pubchem.ncbi.nlm.nih.gov/substance/?source=chemidplus&sourceid=0015964791 | |
Description | ChemIDplus is a free, web search system that provides access to the structure and nomenclature authority files used for the identification of chemical substances cited in National Library of Medicine (NLM) databases, including the TOXNET system. | |
Record name | Benzeneacetic acid, 3,4-dimethoxy-, methyl ester | |
Source | EPA Chemicals under the TSCA | |
URL | https://www.epa.gov/chemicals-under-tsca | |
Description | EPA Chemicals under the Toxic Substances Control Act (TSCA) collection contains information on chemicals and their regulations under TSCA, including non-confidential content from the TSCA Chemical Substance Inventory and Chemical Data Reporting. | |
Record name | Benzeneacetic acid, 3,4-dimethoxy-, methyl ester | |
Source | EPA DSSTox | |
URL | https://comptox.epa.gov/dashboard/DTXSID5065982 | |
Description | DSSTox provides a high quality public chemistry resource for supporting improved predictive toxicology. | |
Record name | Methyl 3,4-dimethoxyphenylacetate | |
Source | European Chemicals Agency (ECHA) | |
URL | https://echa.europa.eu/substance-information/-/substanceinfo/100.036.439 | |
Description | The European Chemicals Agency (ECHA) is an agency of the European Union which is the driving force among regulatory authorities in implementing the EU's groundbreaking chemicals legislation for the benefit of human health and the environment as well as for innovation and competitiveness. | |
Explanation | Use of the information, documents and data from the ECHA website is subject to the terms and conditions of this Legal Notice, and subject to other binding limitations provided for under applicable law, the information, documents and data made available on the ECHA website may be reproduced, distributed and/or used, totally or in part, for non-commercial purposes provided that ECHA is acknowledged as the source: "Source: European Chemicals Agency, http://echa.europa.eu/". Such acknowledgement must be included in each copy of the material. ECHA permits and encourages organisations and individuals to create links to the ECHA website under the following cumulative conditions: Links can only be made to webpages that provide a link to the Legal Notice page. | |
Record name | Methyl (3,4-dimethoxyphenyl)acetate | |
Source | FDA Global Substance Registration System (GSRS) | |
URL | https://gsrs.ncats.nih.gov/ginas/app/beta/substances/GUW5AW3B4Z | |
Description | The FDA Global Substance Registration System (GSRS) enables the efficient and accurate exchange of information on what substances are in regulated products. Instead of relying on names, which vary across regulatory domains, countries, and regions, the GSRS knowledge base makes it possible for substances to be defined by standardized, scientific descriptions. | |
Explanation | Unless otherwise noted, the contents of the FDA website (www.fda.gov), both text and graphics, are not copyrighted. They are in the public domain and may be republished, reprinted and otherwise used freely by anyone without the need to obtain permission from FDA. Credit to the U.S. Food and Drug Administration as the source is appreciated but not required. | |
Synthesis routes and methods I
Procedure details
Synthesis routes and methods II
Procedure details
Synthesis routes and methods III
Procedure details
Synthesis routes and methods IV
Procedure details
Synthesis routes and methods V
Procedure details
Retrosynthesis Analysis
AI-Powered Synthesis Planning: Our tool employs the Template_relevance Pistachio, Template_relevance Bkms_metabolic, Template_relevance Pistachio_ringbreaker, Template_relevance Reaxys, Template_relevance Reaxys_biocatalysis model, leveraging a vast database of chemical reactions to predict feasible synthetic routes.
One-Step Synthesis Focus: Specifically designed for one-step synthesis, it provides concise and direct routes for your target compounds, streamlining the synthesis process.
Accurate Predictions: Utilizing the extensive PISTACHIO, BKMS_METABOLIC, PISTACHIO_RINGBREAKER, REAXYS, REAXYS_BIOCATALYSIS database, our tool offers high-accuracy predictions, reflecting the latest in chemical research and data.
Strategy Settings
Precursor scoring | Relevance Heuristic |
---|---|
Min. plausibility | 0.01 |
Model | Template_relevance |
Template Set | Pistachio/Bkms_metabolic/Pistachio_ringbreaker/Reaxys/Reaxys_biocatalysis |
Top-N result to add to graph | 6 |
Feasible Synthetic Routes
Disclaimer and Information on In-Vitro Research Products
Please be aware that all articles and product information presented on BenchChem are intended solely for informational purposes. The products available for purchase on BenchChem are specifically designed for in-vitro studies, which are conducted outside of living organisms. In-vitro studies, derived from the Latin term "in glass," involve experiments performed in controlled laboratory settings using cells or tissues. It is important to note that these products are not categorized as medicines or drugs, and they have not received approval from the FDA for the prevention, treatment, or cure of any medical condition, ailment, or disease. We must emphasize that any form of bodily introduction of these products into humans or animals is strictly prohibited by law. It is essential to adhere to these guidelines to ensure compliance with legal and ethical standards in research and experimentation.