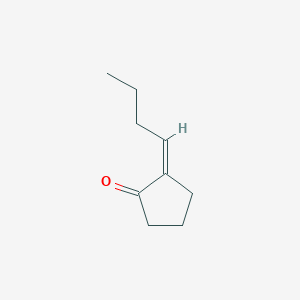
Cyclopentanone, 2-butylidene-
- Click on QUICK INQUIRY to receive a quote from our team of experts.
- With the quality product at a COMPETITIVE price, you can focus more on your research.
Overview
Description
Cyclopentanone, 2-butylidene-, also known as 2-Butylidene-4-cyclopentenone, is a cyclic ketone with a butylidene group attached to the cyclopentenone ring. It has gained significant attention in the scientific community due to its potential applications in various fields.
Mechanism of Action
The mechanism of action of Cyclopentanone, 2-butylidene-, is not fully understood. However, it is believed to exert its biological effects through various pathways such as the inhibition of cyclooxygenase (COX), the induction of apoptosis, and the inhibition of viral replication.
Biochemical and Physiological Effects:
Cyclopentanone, 2-butylidene-, has been shown to possess various biochemical and physiological effects. It has been reported to inhibit the production of pro-inflammatory cytokines such as interleukin-6 (IL-6) and tumor necrosis factor-alpha (TNF-α). It has also been shown to induce apoptosis in cancer cells by activating caspase enzymes.
In addition, Cyclopentanone, 2-butylidene-, has been shown to inhibit viral replication by interfering with the viral life cycle. It has been reported to inhibit the replication of various viruses such as herpes simplex virus (HSV), human immunodeficiency virus (HIV), and influenza virus.
Advantages and Limitations for Lab Experiments
Cyclopentanone, 2-butylidene-, has several advantages and limitations for lab experiments. One of the advantages is its easy synthesis method, which makes it readily available for research purposes. It is also relatively stable and can be easily stored for long periods.
However, one of the limitations is its low solubility in water, which can make it difficult to study its biological effects in aqueous environments. In addition, its toxicity and potential side effects need to be carefully evaluated before using it in lab experiments.
Future Directions
Cyclopentanone, 2-butylidene-, has several potential future directions for research. One of the future directions is the development of new pharmaceuticals based on its structure and biological properties. It can also be used as a starting material for the synthesis of new materials and polymers with unique properties.
In addition, further studies are needed to fully understand its mechanism of action and its potential applications in various fields such as medicine, material science, and agriculture. Furthermore, more research is needed to evaluate its toxicity and potential side effects in vivo.
Conclusion:
Cyclopentanone, 2-butylidene-, is a cyclic ketone with a butylidene group attached to the cyclopentenone ring. It has gained significant attention in the scientific community due to its potential applications in various fields. Its synthesis method, scientific research applications, mechanism of action, biochemical and physiological effects, advantages and limitations for lab experiments, and future directions have been explored in this paper. Further research is needed to fully understand its potential applications and evaluate its safety for use in various fields.
Synthesis Methods
Cyclopentanone, 2-butylidene-, can be synthesized through various methods, including the Claisen-Schmidt condensation reaction. In this reaction, cyclopentanone is reacted with 2-butanone in the presence of a base catalyst such as sodium hydroxide. The resulting product is then purified through distillation or recrystallization to obtain pure Cyclopentanone, 2-butylidene-.
Scientific Research Applications
Cyclopentanone, 2-butylidene-, has been extensively studied for its potential applications in various fields such as medicine, material science, and agriculture. In medicine, it has been shown to possess anti-inflammatory, anticancer, and antiviral properties. It has also been used as a starting material for the synthesis of various pharmaceuticals such as anti-tumor agents.
In material science, Cyclopentanone, 2-butylidene-, has been used as a building block for the synthesis of various polymers and materials such as polyesters, polyamides, and polycarbonates. In agriculture, it has been used as a natural insecticide due to its insecticidal properties.
properties
CAS RN |
16424-32-1 |
---|---|
Molecular Formula |
C9H14O |
Molecular Weight |
138.21 g/mol |
IUPAC Name |
2-butylidenecyclopentan-1-one |
InChI |
InChI=1S/C9H14O/c1-2-3-5-8-6-4-7-9(8)10/h5H,2-4,6-7H2,1H3 |
InChI Key |
PJDOECJDCGNWBG-UHFFFAOYSA-N |
Isomeric SMILES |
CCC/C=C\1/CCCC1=O |
SMILES |
CCCC=C1CCCC1=O |
Canonical SMILES |
CCCC=C1CCCC1=O |
Other CAS RN |
16424-32-1 |
Origin of Product |
United States |
Disclaimer and Information on In-Vitro Research Products
Please be aware that all articles and product information presented on BenchChem are intended solely for informational purposes. The products available for purchase on BenchChem are specifically designed for in-vitro studies, which are conducted outside of living organisms. In-vitro studies, derived from the Latin term "in glass," involve experiments performed in controlled laboratory settings using cells or tissues. It is important to note that these products are not categorized as medicines or drugs, and they have not received approval from the FDA for the prevention, treatment, or cure of any medical condition, ailment, or disease. We must emphasize that any form of bodily introduction of these products into humans or animals is strictly prohibited by law. It is essential to adhere to these guidelines to ensure compliance with legal and ethical standards in research and experimentation.