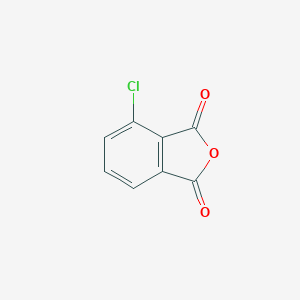
3-Chlorophthalic anhydride
Overview
Description
3-Chlorophthalic anhydride is a chlorinated derivative of phthalic anhydride, which is widely used in the chemical industry. While the provided papers do not directly focus on this compound, they offer insights into the properties and reactivity of similar compounds, such as 4,5-dichlorophthalic anhydride and tetrahalophthalic anhydrides, which can be extrapolated to understand the behavior of this compound .
Synthesis Analysis
The synthesis of chlorinated phthalic anhydrides, like this compound, can be achieved through chlorination reactions of phthalic anhydride or its derivatives. For instance, selective hydrodechlorination of tetrachlorophthalic anhydride can yield this compound as a product . The synthesis process is crucial for the production of intermediates in the chemical and pharmaceutical industries.
Molecular Structure Analysis
The molecular structure of chlorinated phthalic anhydrides is characterized by the presence of halogen atoms, which can influence the electronic distribution and reactivity of the molecule. X-ray diffraction studies of similar compounds have revealed the presence of halogen bonds and interactions between the anhydride oxygen and the π-system of the anhydride ring . These structural features are important for understanding the reactivity and potential applications of this compound.
Chemical Reactions Analysis
Chlorinated phthalic anhydrides, including this compound, can undergo various chemical reactions, such as nucleophilic attack by amines or thiosemicarbazide, leading to the opening of the anhydride ring and the formation of carboxylic acid derivatives . These reactions are significant for the synthesis of a wide range of chemical products.
Physical and Chemical Properties Analysis
The physical and chemical properties of this compound, such as solubility, are influenced by temperature and solvent composition. Studies have shown that the solubility of chlorinated phthalic anhydrides increases with temperature and varies with the solvent used . Understanding these properties is essential for the development of purification methods and the design of crystallization processes for the separation and purification of these compounds .
Scientific Research Applications
Purification and Crystallization : 3-Chlorophthalic anhydride's solubility in various solvents makes it significant for purification and crystallization processes. Tao et al. (2016) determined the solubility in binary solvent mixtures and designed a cooling crystallization process to separate and purify this compound, which is essential for its industrial application in manufacturing high purity substances (Tao et al., 2016).
Solubility and Thermodynamic Studies : Understanding the solubility of this compound in different solvents under various conditions is crucial for its application in chemical processes. Wang et al. (2015) measured its solubility in six pure solvents over a range of temperatures and correlated the data with equations to predict solubility under different conditions. These studies are vital for its application in designing and optimizing chemical processes, including separation techniques and formulation of chemical products (Wang et al., 2015).
Phase Diagrams and Solid-Liquid Equilibrium : The study of phase diagrams and solid-liquid equilibrium involving this compound is crucial for its application in separation processes. Ji et al. (2010) determined the solid-liquid phase equilibrium for the ternary system involving 3-Chlorophthalic acid, 4-Chlorophthalic acid, and water, which is fundamental for preparing this compound from mixtures (Ji et al., 2010).
Synthesis of Derivatives and Fluorescent Labels : this compound serves as a precursor for synthesizing various derivatives, including dyes and labels. Ge et al. (2005) synthesized a mixture of chlorofluorescein by condensing resorcinol with this compound, indicating its use in creating sensitive fluorescent probes for detecting multiple target substances in samples (Ge et al., 2005).
Photopolymerization Reactions : this compound can be used in photopolymerization reactions. Xiao et al. (2014) studied the use of derivatives of this compound combined with various compounds to produce radicals and cations upon exposure to low-intensity blue lights, indicating its potential in photopolymerization processes (Xiao et al., 2014).
Safety and Hazards
Mechanism of Action
Target of Action
3-Chlorophthalic anhydride is a monochlorinated aromatic anhydride . It is primarily used in the production of herbicides and pesticides, intermediates for active pharmaceutical ingredients, and as a monomer for the production of polyimides
Mode of Action
As a derivative of phthalic anhydride, it may share similar chemical properties and reactivity . It may interact with its targets through covalent bonding, leading to changes in the target’s structure and function. More research is needed to fully understand its mode of action.
Biochemical Pathways
Given its use in the production of herbicides, pesticides, and pharmaceutical intermediates, it can be inferred that it may influence a variety of biochemical pathways depending on the specific application .
Action Environment
This compound has a melting point of around 123 °C and may hydrolyze in the presence of water . These properties suggest that its stability, efficacy, and mode of action could be influenced by environmental factors such as temperature and humidity.
properties
IUPAC Name |
4-chloro-2-benzofuran-1,3-dione | |
---|---|---|
Source | PubChem | |
URL | https://pubchem.ncbi.nlm.nih.gov | |
Description | Data deposited in or computed by PubChem | |
InChI |
InChI=1S/C8H3ClO3/c9-5-3-1-2-4-6(5)8(11)12-7(4)10/h1-3H | |
Source | PubChem | |
URL | https://pubchem.ncbi.nlm.nih.gov | |
Description | Data deposited in or computed by PubChem | |
InChI Key |
UERPUZBSSSAZJE-UHFFFAOYSA-N | |
Source | PubChem | |
URL | https://pubchem.ncbi.nlm.nih.gov | |
Description | Data deposited in or computed by PubChem | |
Canonical SMILES |
C1=CC2=C(C(=C1)Cl)C(=O)OC2=O | |
Source | PubChem | |
URL | https://pubchem.ncbi.nlm.nih.gov | |
Description | Data deposited in or computed by PubChem | |
Molecular Formula |
C8H3ClO3 | |
Source | PubChem | |
URL | https://pubchem.ncbi.nlm.nih.gov | |
Description | Data deposited in or computed by PubChem | |
DSSTOX Substance ID |
DTXSID10151653 | |
Record name | 3-Chlorophthalic anhydride | |
Source | EPA DSSTox | |
URL | https://comptox.epa.gov/dashboard/DTXSID10151653 | |
Description | DSSTox provides a high quality public chemistry resource for supporting improved predictive toxicology. | |
Molecular Weight |
182.56 g/mol | |
Source | PubChem | |
URL | https://pubchem.ncbi.nlm.nih.gov | |
Description | Data deposited in or computed by PubChem | |
CAS RN |
117-21-5 | |
Record name | 3-Chlorophthalic anhydride | |
Source | CAS Common Chemistry | |
URL | https://commonchemistry.cas.org/detail?cas_rn=117-21-5 | |
Description | CAS Common Chemistry is an open community resource for accessing chemical information. Nearly 500,000 chemical substances from CAS REGISTRY cover areas of community interest, including common and frequently regulated chemicals, and those relevant to high school and undergraduate chemistry classes. This chemical information, curated by our expert scientists, is provided in alignment with our mission as a division of the American Chemical Society. | |
Explanation | The data from CAS Common Chemistry is provided under a CC-BY-NC 4.0 license, unless otherwise stated. | |
Record name | 3-Chlorophthalic anhydride | |
Source | ChemIDplus | |
URL | https://pubchem.ncbi.nlm.nih.gov/substance/?source=chemidplus&sourceid=0000117215 | |
Description | ChemIDplus is a free, web search system that provides access to the structure and nomenclature authority files used for the identification of chemical substances cited in National Library of Medicine (NLM) databases, including the TOXNET system. | |
Record name | 3-Chlorophthalic anhydride | |
Source | DTP/NCI | |
URL | https://dtp.cancer.gov/dtpstandard/servlet/dwindex?searchtype=NSC&outputformat=html&searchlist=122937 | |
Description | The NCI Development Therapeutics Program (DTP) provides services and resources to the academic and private-sector research communities worldwide to facilitate the discovery and development of new cancer therapeutic agents. | |
Explanation | Unless otherwise indicated, all text within NCI products is free of copyright and may be reused without our permission. Credit the National Cancer Institute as the source. | |
Record name | 1,3-Isobenzofurandione, 4-chloro- | |
Source | EPA Chemicals under the TSCA | |
URL | https://www.epa.gov/chemicals-under-tsca | |
Description | EPA Chemicals under the Toxic Substances Control Act (TSCA) collection contains information on chemicals and their regulations under TSCA, including non-confidential content from the TSCA Chemical Substance Inventory and Chemical Data Reporting. | |
Record name | 3-Chlorophthalic anhydride | |
Source | EPA DSSTox | |
URL | https://comptox.epa.gov/dashboard/DTXSID10151653 | |
Description | DSSTox provides a high quality public chemistry resource for supporting improved predictive toxicology. | |
Record name | 3-chlorophthalic anhydride | |
Source | European Chemicals Agency (ECHA) | |
URL | https://echa.europa.eu/substance-information/-/substanceinfo/100.003.800 | |
Description | The European Chemicals Agency (ECHA) is an agency of the European Union which is the driving force among regulatory authorities in implementing the EU's groundbreaking chemicals legislation for the benefit of human health and the environment as well as for innovation and competitiveness. | |
Explanation | Use of the information, documents and data from the ECHA website is subject to the terms and conditions of this Legal Notice, and subject to other binding limitations provided for under applicable law, the information, documents and data made available on the ECHA website may be reproduced, distributed and/or used, totally or in part, for non-commercial purposes provided that ECHA is acknowledged as the source: "Source: European Chemicals Agency, http://echa.europa.eu/". Such acknowledgement must be included in each copy of the material. ECHA permits and encourages organisations and individuals to create links to the ECHA website under the following cumulative conditions: Links can only be made to webpages that provide a link to the Legal Notice page. | |
Record name | 3-CHLOROPHTHALIC ANHYDRIDE | |
Source | FDA Global Substance Registration System (GSRS) | |
URL | https://gsrs.ncats.nih.gov/ginas/app/beta/substances/VO7NXT0137 | |
Description | The FDA Global Substance Registration System (GSRS) enables the efficient and accurate exchange of information on what substances are in regulated products. Instead of relying on names, which vary across regulatory domains, countries, and regions, the GSRS knowledge base makes it possible for substances to be defined by standardized, scientific descriptions. | |
Explanation | Unless otherwise noted, the contents of the FDA website (www.fda.gov), both text and graphics, are not copyrighted. They are in the public domain and may be republished, reprinted and otherwise used freely by anyone without the need to obtain permission from FDA. Credit to the U.S. Food and Drug Administration as the source is appreciated but not required. | |
Synthesis routes and methods I
Procedure details
Synthesis routes and methods II
Procedure details
Synthesis routes and methods III
Procedure details
Synthesis routes and methods IV
Procedure details
Retrosynthesis Analysis
AI-Powered Synthesis Planning: Our tool employs the Template_relevance Pistachio, Template_relevance Bkms_metabolic, Template_relevance Pistachio_ringbreaker, Template_relevance Reaxys, Template_relevance Reaxys_biocatalysis model, leveraging a vast database of chemical reactions to predict feasible synthetic routes.
One-Step Synthesis Focus: Specifically designed for one-step synthesis, it provides concise and direct routes for your target compounds, streamlining the synthesis process.
Accurate Predictions: Utilizing the extensive PISTACHIO, BKMS_METABOLIC, PISTACHIO_RINGBREAKER, REAXYS, REAXYS_BIOCATALYSIS database, our tool offers high-accuracy predictions, reflecting the latest in chemical research and data.
Strategy Settings
Precursor scoring | Relevance Heuristic |
---|---|
Min. plausibility | 0.01 |
Model | Template_relevance |
Template Set | Pistachio/Bkms_metabolic/Pistachio_ringbreaker/Reaxys/Reaxys_biocatalysis |
Top-N result to add to graph | 6 |
Feasible Synthetic Routes
Q & A
Q1: What is the molecular formula and weight of 3-chlorophthalic anhydride?
A1: this compound has the molecular formula C8H3ClO3 and a molecular weight of 182.56 g/mol.
Q2: What makes this compound useful in synthesis?
A2: The anhydride group in this compound is highly reactive towards nucleophiles like amines, allowing for the formation of imide bonds. This makes it a valuable precursor for synthesizing various compounds, including polyimides and other high-performance polymers .
Q3: How does the solubility of this compound vary with solvents and temperature?
A3: Research indicates that this compound exhibits varying solubility in different solvents. Its solubility increases with temperature in solvents like ethyl acetate, acetone, 1,4-dioxane , tert-amyl alcohol, carbon tetrachloride, cyclohexane, methanol, methyl acetate, and acetonitrile . Understanding this solubility behavior is crucial for designing separation and purification processes.
Q4: What are some specific applications of this compound?
A4: this compound serves as a crucial starting material in the synthesis of various compounds. For example, it's used in the laboratory synthesis of mercury-free 1-chloroanthraquinone . It's also a key component in the production of high-performance polymers, such as polyimides, known for their excellent thermal stability, mechanical strength, and chemical resistance .
Q5: How is 3-fluorophthalic anhydride synthesized from this compound?
A5: Two main methods utilize this compound for 3-fluorophthalic anhydride synthesis:
- Homogeneous Fluorination: This method utilizes an organic complex of hydrogen fluoride as a fluorination reagent to perform a homogeneous fluorination reaction on this compound .
- Phase Transfer Catalysis: This approach involves reacting this compound with anhydrous potassium fluoride in a solvent, using a phase transfer catalyst . This method offers advantages like a short process route, operational flexibility, and cost-effectiveness.
Q6: Can you elaborate on the use of this compound in synthesizing thioether dianhydrides?
A6: this compound, alongside 4-chlorophthalic anhydride, is crucial for producing various isomeric diphenylthioether dianhydrides. These dianhydrides, including 3,3',4,4'-diphenylthioether dianhydride (4,4'-TDPA), 2,3,3',4'-diphenylthioether dianhydride (3,4'-TDPA), and 2,2',3,3'-diphenylthioether dianhydride (3,3'-TDPA), are subsequently used to create polyimides with distinct properties . A simplified, environmentally friendly method has been developed using a two-pot process for this synthesis, showcasing the potential for greener chemical production .
Q7: Why is understanding the solid-liquid equilibrium of systems containing this compound important?
A7: Knowledge of the solid-liquid equilibrium is crucial for developing efficient separation and purification techniques for this compound. This understanding aids in designing crystallization processes, which are energy-efficient and yield high-purity products.
Q8: What is the significance of the ternary phase diagram for the 3-chlorophthalic acid + 4-chlorophthalic acid + water system?
A8: The ternary phase diagram provides crucial information on the solubility of both 3-chlorophthalic acid and 4-chlorophthalic acid in water at various temperatures . This information is paramount for developing crystallization-based separation methods for these compounds. The phase diagram reveals the presence of an adductive compound (3-C6H3Cl(COOH)2 ·4- C6H3Cl(COOH)2) and highlights the influence of temperature on the crystallization fields of different components.
Q9: How can this compound and 4-chlorophthalic anhydride be separated?
A9: Studying ternary systems, such as the one with ethyl acetate or acetone as a solvent, provides insights into their separation. These systems exhibit two pure solid phases: pure 4-chlorophthalic anhydride and pure this compound. By manipulating temperature and solvent composition, crystallization can be directed to preferentially crystallize one isomer, enabling their separation.
Q10: How can monochlorophthalic anhydride and monochlorophthalic acid be analyzed in substrates?
A10: A high-performance liquid chromatography (HPLC) method offers a solution for the simultaneous determination of this compound, 4-chlorophthalic anhydride, 3-chlorophthalic acid, and 4-chlorophthalic acid . This method involves esterification with ethanol, converting the anhydrides into monochloro-O-carboxyl ethyl benzoate isomers while leaving the acids unaffected.
Disclaimer and Information on In-Vitro Research Products
Please be aware that all articles and product information presented on BenchChem are intended solely for informational purposes. The products available for purchase on BenchChem are specifically designed for in-vitro studies, which are conducted outside of living organisms. In-vitro studies, derived from the Latin term "in glass," involve experiments performed in controlled laboratory settings using cells or tissues. It is important to note that these products are not categorized as medicines or drugs, and they have not received approval from the FDA for the prevention, treatment, or cure of any medical condition, ailment, or disease. We must emphasize that any form of bodily introduction of these products into humans or animals is strictly prohibited by law. It is essential to adhere to these guidelines to ensure compliance with legal and ethical standards in research and experimentation.