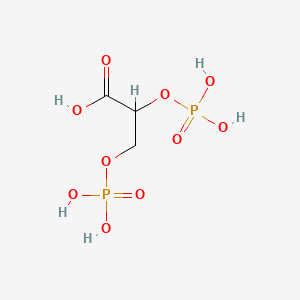
2,3-Diphosphoglyceric acid
Overview
Description
2,3-Diphosphoglyceric acid (2,3-DPG) is an intermediate of the glycolytic pathway . It allosterically binds to deoxygenated hemoglobin, stabilizing it and facilitating oxygen release at tissue sites . This binding decreases hemoglobin’s oxygen affinity, promoting oxygen delivery to tissues .
Synthesis Analysis
The formation of 2,3-BPG is a result of the isomerization of 1,3-BPG, a process catalyzed by the enzyme BPG mutase . This compound is then broken down by 2,3-BPG phosphatase to form 3-PGA (3-phosphoglycerate) . Both the synthesis and breakdown of 2,3-BPG are part of the glycolytic pathway .Molecular Structure Analysis
The molecular formula of 2,3-DPG is C3H8O10P2 . Its average mass is 266.037 Da and its monoisotopic mass is 265.959259 Da .Chemical Reactions Analysis
The formation of 2,3-BPG is a result of the isomerization of 1,3-BPG, a process catalyzed by the enzyme BPG mutase . This compound is then broken down by 2,3-BPG phosphatase to form 3-PGA (3-phosphoglycerate) .Physical And Chemical Properties Analysis
2,3-DPG has a density of 2.1±0.1 g/cm^3 . Its boiling point is 669.8±65.0 °C at 760 mmHg . The enthalpy of vaporization is 107.3±6.0 kJ/mol . The flash point is 358.9±34.3 °C . The index of refraction is 1.572 .Scientific Research Applications
Hemoglobin Allosteric Regulation
2,3-DPG is a cofactor that interacts with hemoglobin (Hb) within red blood cells (erythrocytes). It binds to deoxygenated Hb, reducing its affinity for oxygen. This allosteric effect ensures efficient oxygen release in tissues, especially during hypoxia (low oxygen levels) .
Blood Gas Transport
In erythrocytes, 2,3-DPG modulates the oxygen dissociation curve of Hb. By promoting oxygen release in peripheral tissues, it enhances oxygen transport from the lungs to cells throughout the body .
Clinical Diagnostics
Measurement of 2,3-DPG levels in blood provides insights into erythrocyte metabolism and oxygen transport. Abnormal levels may indicate certain medical conditions, such as anemia or hemoglobinopathies .
Biochemical Reference Compound
Researchers use 2,3-DPG as a reference compound in studies related to glycolysis, erythrocyte metabolism, and Hb function. Its stable structure and well-characterized properties make it valuable for calibration and comparison .
Phosphoglycerate Mutase Activity
2,3-DPG serves as a cofactor for phosphoglycerate mutase, an enzyme involved in glycolysis. It facilitates the conversion of 3-phosphoglycerate to 2-phosphoglycerate, a critical step in energy production .
Blood Storage and Transfusion
During blood storage for transfusion, 2,3-DPG levels decrease. Understanding its role helps optimize blood storage conditions to maintain oxygen-carrying capacity .
Mechanism of Action
Target of Action
The primary target of 2,3-diphosphoglyceric acid (2,3-DPG) is hemoglobin, specifically deoxygenated hemoglobin . Hemoglobin is a protein in red blood cells that carries oxygen from the lungs to the body’s tissues .
Mode of Action
2,3-DPG allosterically binds to deoxygenated hemoglobin, stabilizing it and facilitating oxygen release at tissue sites . This binding decreases hemoglobin’s oxygen affinity, promoting oxygen delivery to tissues .
Biochemical Pathways
2,3-DPG is an intermediate of the glycolytic pathway . It is formed as a result of the isomerization of 1,3-bisphosphoglycerate, a process catalyzed by the enzyme BPG mutase . This compound is then broken down by 2,3-BPG phosphatase to form 3-phosphoglycerate . Both the synthesis and breakdown of 2,3-DPG are part of the glycolytic pathway .
Pharmacokinetics
It is known that the rate of 2,3-dpg degradation is accelerated when the cellular level of phosphoglycolate is increased by incubation with exogenous glycolate . This suggests that 2,3-DPG content in erythrocytes can be directly regulated through modulation of phosphatase/synthase activities .
Result of Action
The binding of 2,3-DPG to deoxygenated hemoglobin results in a rightward shift of the oxygen dissociation curve . This means that at any given partial pressure of oxygen, hemoglobin has a lower affinity for oxygen, allowing more oxygen to be released to the tissues . This is particularly beneficial in conditions where tissue oxygen demand is high .
Action Environment
Environmental factors such as hypoxia can influence the action of 2,3-DPG. For example, intense exercise in hypoxic conditions leads to a decrease in 2,3-DPG concentration, primarily due to exercise-induced acidosis . This suggests that the action, efficacy, and stability of 2,3-DPG can be influenced by the oxygen levels in the environment .
Future Directions
2,3-DPG has been used in the study of Alzheimer’s disease (AD) . It is also being investigated for its potential in enhancing oxygen delivery by human erythrocytes .
Relevant Papers Several papers have been published on 2,3-DPG. One study analyzed the effect of prolonged intense exercise under normoxic and hypoxic conditions on 2,3-DPG level in cyclists . Another study conducted a computational analysis of the interaction of aluminum with 2,3-DPG . A third study investigated the potential of targeting the DPG synthase/phosphatase enzyme system to increase DPG levels in the cell .
properties
IUPAC Name |
2,3-diphosphonooxypropanoic acid | |
---|---|---|
Source | PubChem | |
URL | https://pubchem.ncbi.nlm.nih.gov | |
Description | Data deposited in or computed by PubChem | |
InChI |
InChI=1S/C3H8O10P2/c4-3(5)2(13-15(9,10)11)1-12-14(6,7)8/h2H,1H2,(H,4,5)(H2,6,7,8)(H2,9,10,11) | |
Source | PubChem | |
URL | https://pubchem.ncbi.nlm.nih.gov | |
Description | Data deposited in or computed by PubChem | |
InChI Key |
XOHUEYCVLUUEJJ-UHFFFAOYSA-N | |
Source | PubChem | |
URL | https://pubchem.ncbi.nlm.nih.gov | |
Description | Data deposited in or computed by PubChem | |
Canonical SMILES |
C(C(C(=O)O)OP(=O)(O)O)OP(=O)(O)O | |
Source | PubChem | |
URL | https://pubchem.ncbi.nlm.nih.gov | |
Description | Data deposited in or computed by PubChem | |
Molecular Formula |
C3H8O10P2 | |
Source | PubChem | |
URL | https://pubchem.ncbi.nlm.nih.gov | |
Description | Data deposited in or computed by PubChem | |
DSSTOX Substance ID |
DTXSID10861801 | |
Record name | Diphosphoglyceric acid | |
Source | EPA DSSTox | |
URL | https://comptox.epa.gov/dashboard/DTXSID10861801 | |
Description | DSSTox provides a high quality public chemistry resource for supporting improved predictive toxicology. | |
Molecular Weight |
266.04 g/mol | |
Source | PubChem | |
URL | https://pubchem.ncbi.nlm.nih.gov | |
Description | Data deposited in or computed by PubChem | |
Product Name |
2,3-Diphosphoglyceric acid | |
CAS RN |
138-81-8 | |
Record name | Diphosphoglyceric acid | |
Source | CAS Common Chemistry | |
URL | https://commonchemistry.cas.org/detail?cas_rn=138-81-8 | |
Description | CAS Common Chemistry is an open community resource for accessing chemical information. Nearly 500,000 chemical substances from CAS REGISTRY cover areas of community interest, including common and frequently regulated chemicals, and those relevant to high school and undergraduate chemistry classes. This chemical information, curated by our expert scientists, is provided in alignment with our mission as a division of the American Chemical Society. | |
Explanation | The data from CAS Common Chemistry is provided under a CC-BY-NC 4.0 license, unless otherwise stated. | |
Record name | 2,3-Diphosphoglycerate | |
Source | ChemIDplus | |
URL | https://pubchem.ncbi.nlm.nih.gov/substance/?source=chemidplus&sourceid=0000138818 | |
Description | ChemIDplus is a free, web search system that provides access to the structure and nomenclature authority files used for the identification of chemical substances cited in National Library of Medicine (NLM) databases, including the TOXNET system. | |
Record name | Diphosphoglyceric acid | |
Source | EPA DSSTox | |
URL | https://comptox.epa.gov/dashboard/DTXSID10861801 | |
Description | DSSTox provides a high quality public chemistry resource for supporting improved predictive toxicology. | |
Record name | 2,3-DIPHOSPHOGLYCERATE | |
Source | FDA Global Substance Registration System (GSRS) | |
URL | https://gsrs.ncats.nih.gov/ginas/app/beta/substances/TZ4454O4YZ | |
Description | The FDA Global Substance Registration System (GSRS) enables the efficient and accurate exchange of information on what substances are in regulated products. Instead of relying on names, which vary across regulatory domains, countries, and regions, the GSRS knowledge base makes it possible for substances to be defined by standardized, scientific descriptions. | |
Explanation | Unless otherwise noted, the contents of the FDA website (www.fda.gov), both text and graphics, are not copyrighted. They are in the public domain and may be republished, reprinted and otherwise used freely by anyone without the need to obtain permission from FDA. Credit to the U.S. Food and Drug Administration as the source is appreciated but not required. | |
Retrosynthesis Analysis
AI-Powered Synthesis Planning: Our tool employs the Template_relevance Pistachio, Template_relevance Bkms_metabolic, Template_relevance Pistachio_ringbreaker, Template_relevance Reaxys, Template_relevance Reaxys_biocatalysis model, leveraging a vast database of chemical reactions to predict feasible synthetic routes.
One-Step Synthesis Focus: Specifically designed for one-step synthesis, it provides concise and direct routes for your target compounds, streamlining the synthesis process.
Accurate Predictions: Utilizing the extensive PISTACHIO, BKMS_METABOLIC, PISTACHIO_RINGBREAKER, REAXYS, REAXYS_BIOCATALYSIS database, our tool offers high-accuracy predictions, reflecting the latest in chemical research and data.
Strategy Settings
Precursor scoring | Relevance Heuristic |
---|---|
Min. plausibility | 0.01 |
Model | Template_relevance |
Template Set | Pistachio/Bkms_metabolic/Pistachio_ringbreaker/Reaxys/Reaxys_biocatalysis |
Top-N result to add to graph | 6 |
Feasible Synthetic Routes
Q & A
Q1: How does 2,3-DPG interact with hemoglobin and affect oxygen binding?
A1: 2,3-DPG binds to a specific site on deoxyhemoglobin, located between the β-polypeptide chains [, ]. This binding stabilizes the deoxy configuration of hemoglobin, which has a lower affinity for oxygen. As a result, 2,3-DPG promotes the release of oxygen from hemoglobin to the tissues.
Q2: What physiological conditions lead to changes in 2,3-DPG levels, and how do these changes impact oxygen delivery?
A2: Conditions like hypoxia (low oxygen levels) stimulate 2,3-DPG synthesis in red blood cells []. This increase in 2,3-DPG shifts the oxygen-hemoglobin dissociation curve to the right, facilitating oxygen release to tissues where it is needed most []. Conversely, conditions like chronic alcoholism can lead to elevated 2,3-DPG levels even in the absence of hypoxia [].
Q3: How does the sensitivity of different animal hemoglobins to 2,3-DPG vary, and what is the evolutionary significance of this variation?
A3: Sensitivity to 2,3-DPG varies among species, with larger animals generally exhibiting higher sensitivity []. This variation is attributed to differences in the amino acid composition of hemoglobin, particularly charged residues. The selection for these charged residues in mammals with higher metabolic rates suggests a positive Darwinian selection for efficient oxygen transport [].
Q4: What is the molecular formula and weight of 2,3-DPG?
A4: The molecular formula of 2,3-DPG is C6H10O12P2, and its molecular weight is 356.07 g/mol.
Q5: How does the stability of the oxygen-hemoglobin dissociation curve in stored blood relate to cell packing?
A5: Research suggests that the stability of the oxygen-hemoglobin dissociation curve in stored blood is influenced by the area of the cell-plasma interface. Factors like the orientation of collection tubes and storage container size can impact cell packing and subsequently affect the dissociation curve's stability [].
Q6: What is the role of 2,3-DPG in the phosphoglycerate mutase reaction?
A6: 2,3-DPG acts as a cofactor for the enzyme phosphoglycerate mutase (PGM) [, ]. PGM catalyzes the interconversion of 3-phosphoglyceric acid (3-PGA) and 2-phosphoglyceric acid (2-PGA), a crucial step in glycolysis. 2,3-DPG is essential for the enzyme's activity, particularly in the type of PGM found in muscle tissue.
Q7: Can 2,3-DPG levels be manipulated to enhance oxygen delivery?
A7: Studies have shown that inhibiting pyruvate kinase, an enzyme upstream in the glycolytic pathway, leads to increased 3-PGA levels in red blood cells. This increase in 3-PGA enhances 2,3-DPG synthesis, thereby increasing oxygen delivery [].
Q8: Have computational studies been conducted to understand the interaction of 2,3-DPG with aluminum?
A8: Yes, density functional theory (DFT) calculations have been used to investigate the affinity of aluminum for 2,3-DPG. These studies suggest that 2,3-DPG can compete with citrate for binding to aluminum at physiological pH, highlighting the potential for aluminum to interfere with 2,3-DPG's role in oxygen transport [].
Q9: Have any structural analogues of 2,3-DPG been synthesized, and what are their effects on hemoglobin oxygen affinity?
A9: Yes, bisphosphates of ethylene glycol and 1,2-propanediol, structural analogues of 2,3-DPG, have been synthesized and shown to reduce the oxygen affinity of hemoglobin []. This finding underscores the importance of the phosphate groups and the overall structure of 2,3-DPG for its biological activity.
Disclaimer and Information on In-Vitro Research Products
Please be aware that all articles and product information presented on BenchChem are intended solely for informational purposes. The products available for purchase on BenchChem are specifically designed for in-vitro studies, which are conducted outside of living organisms. In-vitro studies, derived from the Latin term "in glass," involve experiments performed in controlled laboratory settings using cells or tissues. It is important to note that these products are not categorized as medicines or drugs, and they have not received approval from the FDA for the prevention, treatment, or cure of any medical condition, ailment, or disease. We must emphasize that any form of bodily introduction of these products into humans or animals is strictly prohibited by law. It is essential to adhere to these guidelines to ensure compliance with legal and ethical standards in research and experimentation.