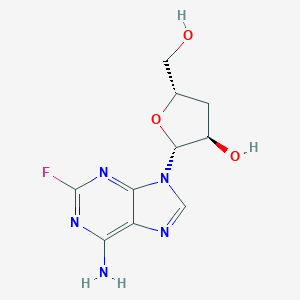
2-Fluoro-3'-deoxyadenosine
Overview
Description
2-Fluoro-3’-deoxyadenosine is a modified nucleoside analog where the hydroxyl group at the 3’ position of the ribose sugar is replaced by a fluorine atom. This modification imparts unique properties to the compound, making it valuable in various scientific and medical applications. It is structurally similar to 2’-deoxyadenosine, a natural component of DNA, but with enhanced stability and altered biological activity due to the presence of the fluorine atom.
Preparation Methods
Synthetic Routes and Reaction Conditions: The synthesis of 2-Fluoro-3’-deoxyadenosine typically involves the fluorination of 2’-deoxyadenosine. One common method includes the use of diethylaminosulfur trifluoride (DAST) as a fluorinating agent. The reaction is carried out under anhydrous conditions to prevent hydrolysis of the intermediate products. The reaction proceeds as follows:
- 2’-Deoxyadenosine is dissolved in an anhydrous solvent such as dichloromethane.
- DAST is added dropwise to the solution at low temperatures (0-5°C).
- The reaction mixture is stirred for several hours, gradually warming to room temperature.
- The product is purified using column chromatography to obtain pure 2-Fluoro-3’-deoxyadenosine.
Industrial Production Methods: Industrial production of 2-Fluoro-3’-deoxyadenosine may involve enzymatic synthesis using purine nucleoside phosphorylases. This method is advantageous due to its high specificity and mild reaction conditions. The enzymatic process involves:
- Using recombinant Escherichia coli strains expressing purine nucleoside phosphorylase.
- Incubating the bacterial culture with 2’-deoxyadenosine and a fluorine donor.
- Isolating and purifying the product through filtration and chromatography.
Chemical Reactions Analysis
Types of Reactions: 2-Fluoro-3’-deoxyadenosine undergoes various chemical reactions, including:
Substitution Reactions: The fluorine atom can be replaced by other nucleophiles under specific conditions.
Oxidation and Reduction: The compound can participate in redox reactions, altering its oxidation state.
Hydrolysis: Under acidic or basic conditions, the glycosidic bond can be cleaved, releasing the base and sugar components.
Common Reagents and Conditions:
Substitution: Nucleophiles such as thiols or amines can replace the fluorine atom in the presence of a suitable catalyst.
Oxidation: Oxidizing agents like potassium permanganate or hydrogen peroxide can oxidize the compound.
Reduction: Reducing agents such as sodium borohydride can reduce the compound to its corresponding alcohol.
Major Products:
Substitution: Products include thiolated or aminated derivatives.
Oxidation: Oxidized products may include carboxylic acids or aldehydes.
Reduction: Reduced products include alcohols or alkanes.
Scientific Research Applications
Scientific Research Applications
1.1 Chemistry
- Building Block for Oligonucleotides : 2-Fluoro-3'-deoxyadenosine is utilized as a precursor for synthesizing modified oligonucleotides and nucleic acids. Its structural similarity to natural nucleosides allows it to be incorporated into DNA, facilitating studies on nucleic acid behavior and function.
1.2 Biology
- DNA Replication and Repair Studies : Researchers employ this compound to investigate mechanisms of DNA replication and repair. Its incorporation into DNA strands can disrupt normal polymerase activity, providing insights into the fidelity of DNA synthesis and the cellular response to DNA damage .
Medical Applications
2.1 Antiviral and Anticancer Agent
- Cytotoxic Properties : this compound has been studied as a potential prodrug for 2-fluoroadenine, which exhibits significant cytotoxicity against various tumor cells. The compound acts by inhibiting DNA synthesis, making it effective against rapidly dividing cancer cells .
- Mechanism of Action : Upon incorporation into DNA, it leads to chain termination during replication, effectively halting cell proliferation. Additionally, it targets purine nucleoside phosphorylase, an enzyme critical for nucleotide metabolism, further enhancing its cytotoxic effects .
2.2 Therapeutic Potential in Infectious Diseases
- Preclinical Studies : Research indicates that this compound demonstrates potent activity against Trypanosoma brucei, the causative agent of African trypanosomiasis. In vitro studies show significant trypanocidal effects, and in vivo studies confirm its efficacy in curing infections in animal models .
Insights from Case Studies
4.1 Clinical Trials and Drug Development
Numerous fluorinated nucleosides have progressed into clinical trials or received FDA approval for therapeutic use, highlighting the significance of this compound class in drug discovery . For instance, drugs like azvudine have shown promise in treating viral infections such as COVID-19 and HIV, underscoring the therapeutic potential of fluorinated nucleosides including 2-fluoro derivatives .
Mechanism of Action
The mechanism of action of 2-Fluoro-3’-deoxyadenosine involves its incorporation into DNA during replication. Once incorporated, it disrupts the normal function of DNA polymerases, leading to chain termination and inhibition of DNA synthesis. This results in the inhibition of cell proliferation, making it effective against rapidly dividing cells such as cancer cells. The compound also targets purine nucleoside phosphorylase, an enzyme involved in nucleotide metabolism, further contributing to its cytotoxic effects.
Comparison with Similar Compounds
2-Fluoro-3’-deoxyadenosine can be compared with other fluorinated nucleoside analogs such as:
2-Fluoro-2’-deoxycytidine: Similar in structure but with a cytosine base instead of adenine. It is used as an antiviral agent.
2-Fluoro-2’-deoxyguanosine: Contains a guanine base and is studied for its potential in cancer therapy.
2-Fluoro-2’-deoxyuridine: Contains a uracil base and is used in the treatment of certain cancers.
Uniqueness: The uniqueness of 2-Fluoro-3’-deoxyadenosine lies in its specific targeting of DNA synthesis and purine nucleoside phosphorylase, making it a potent agent for cancer therapy. Its structural modification with fluorine enhances its stability and bioavailability compared to other nucleoside analogs.
Biological Activity
2-Fluoro-3'-deoxyadenosine (2-F-3'-dA), also known as 2-fluorocordycepin, is a modified nucleoside analog where the hydroxyl group at the 3' position of the ribose sugar is replaced by a fluorine atom. This modification enhances its stability and alters its biological activity, making it a compound of interest in various scientific and medical fields, particularly in oncology and virology.
This compound has the following chemical characteristics:
- Molecular Formula: C10H12FN5O3
- Molecular Weight: 253.23 g/mol
- CAS Number: 15386-69-3
This compound's structural modification allows it to resist degradation by adenosine deaminase (ADA), which is significant for its therapeutic applications, particularly in cancer treatment and antiviral therapies .
The biological activity of 2-F-3'-dA primarily arises from its intracellular phosphorylation to its active triphosphate form (2-F-3'-dATP). This active metabolite can competitively inhibit viral polymerases and interfere with nucleic acid synthesis. The mechanism can be summarized as follows:
- Uptake: The compound enters cells via specific transporters, such as the high-affinity TbAT1/P2 adenosine transporter in Trypanosoma brucei.
- Phosphorylation: Inside the cell, it is phosphorylated by kinases, trapping it within the cell and allowing it to disrupt metabolic processes.
- Nucleic Acid Interference: The phosphorylated form interferes with nucleic acid synthesis, leading to cell death in susceptible organisms or cancer cells .
Antiviral Properties
Research indicates that 2-F-3'-dA exhibits potent antiviral activity. It acts as a prodrug for 2-fluoroadenine, which has demonstrated cytotoxic effects against various viral infections. Its mechanism involves inhibiting viral replication by targeting virus-specific polymerases, thereby preventing further nucleic acid chain elongation .
Anticancer Activity
2-F-3'-dA has shown promise as an anticancer agent through several mechanisms:
- Induction of Apoptosis: The compound has been observed to induce apoptosis in various cancer cell lines, including lung cancer (A549) and leukemia cells (U937). This effect is mediated through pathways involving adenosine receptors and death receptors .
- Inhibition of Tumor Growth: In preclinical studies, 2-F-3'-dA exhibited significant tumor growth inhibition in xenograft models of solid tumors. It has been evaluated in clinical trials for patients with advanced solid tumors .
Comparative Analysis with Other Nucleoside Analogs
Compound Name | Base Type | Mechanism of Action | Key Applications |
---|---|---|---|
This compound | Adenine | Inhibits nucleic acid synthesis | Antiviral, Anticancer |
2-Fluoro-2'-deoxycytidine | Cytosine | Antiviral agent | HIV treatment |
2-Fluoro-2'-deoxyguanosine | Guanine | Cancer therapy | Antitumor |
2-Fluoro-2'-deoxyuridine | Uracil | Cancer treatment | Chemotherapy |
Case Studies
Recent studies have highlighted the efficacy of 2-F-3'-dA in treating Trypanosoma brucei, the causative agent of African sleeping sickness. In vitro studies demonstrated that this compound effectively reduced parasite viability by interfering with essential metabolic processes after being phosphorylated within the parasite .
Additionally, clinical trials involving patients with refractory leukemias have shown promising results, indicating that 2-F-3'-dA can enhance intracellular levels of its active metabolite, thereby improving therapeutic outcomes .
Properties
IUPAC Name |
(2R,3R,5S)-2-(6-amino-2-fluoropurin-9-yl)-5-(hydroxymethyl)oxolan-3-ol | |
---|---|---|
Source | PubChem | |
URL | https://pubchem.ncbi.nlm.nih.gov | |
Description | Data deposited in or computed by PubChem | |
InChI |
InChI=1S/C10H12FN5O3/c11-10-14-7(12)6-8(15-10)16(3-13-6)9-5(18)1-4(2-17)19-9/h3-5,9,17-18H,1-2H2,(H2,12,14,15)/t4-,5+,9+/m0/s1 | |
Source | PubChem | |
URL | https://pubchem.ncbi.nlm.nih.gov | |
Description | Data deposited in or computed by PubChem | |
InChI Key |
PCVQISHHKVORII-OBXARNEKSA-N | |
Source | PubChem | |
URL | https://pubchem.ncbi.nlm.nih.gov | |
Description | Data deposited in or computed by PubChem | |
Canonical SMILES |
C1C(OC(C1O)N2C=NC3=C(N=C(N=C32)F)N)CO | |
Source | PubChem | |
URL | https://pubchem.ncbi.nlm.nih.gov | |
Description | Data deposited in or computed by PubChem | |
Isomeric SMILES |
C1[C@H](O[C@H]([C@@H]1O)N2C=NC3=C(N=C(N=C32)F)N)CO | |
Source | PubChem | |
URL | https://pubchem.ncbi.nlm.nih.gov | |
Description | Data deposited in or computed by PubChem | |
Molecular Formula |
C10H12FN5O3 | |
Source | PubChem | |
URL | https://pubchem.ncbi.nlm.nih.gov | |
Description | Data deposited in or computed by PubChem | |
DSSTOX Substance ID |
DTXSID30432315 | |
Record name | 3'-desoxy-2-fluoradenosin | |
Source | EPA DSSTox | |
URL | https://comptox.epa.gov/dashboard/DTXSID30432315 | |
Description | DSSTox provides a high quality public chemistry resource for supporting improved predictive toxicology. | |
Molecular Weight |
269.23 g/mol | |
Source | PubChem | |
URL | https://pubchem.ncbi.nlm.nih.gov | |
Description | Data deposited in or computed by PubChem | |
CAS No. |
15386-69-3 | |
Record name | 3'-desoxy-2-fluoradenosin | |
Source | EPA DSSTox | |
URL | https://comptox.epa.gov/dashboard/DTXSID30432315 | |
Description | DSSTox provides a high quality public chemistry resource for supporting improved predictive toxicology. | |
Retrosynthesis Analysis
AI-Powered Synthesis Planning: Our tool employs the Template_relevance Pistachio, Template_relevance Bkms_metabolic, Template_relevance Pistachio_ringbreaker, Template_relevance Reaxys, Template_relevance Reaxys_biocatalysis model, leveraging a vast database of chemical reactions to predict feasible synthetic routes.
One-Step Synthesis Focus: Specifically designed for one-step synthesis, it provides concise and direct routes for your target compounds, streamlining the synthesis process.
Accurate Predictions: Utilizing the extensive PISTACHIO, BKMS_METABOLIC, PISTACHIO_RINGBREAKER, REAXYS, REAXYS_BIOCATALYSIS database, our tool offers high-accuracy predictions, reflecting the latest in chemical research and data.
Strategy Settings
Precursor scoring | Relevance Heuristic |
---|---|
Min. plausibility | 0.01 |
Model | Template_relevance |
Template Set | Pistachio/Bkms_metabolic/Pistachio_ringbreaker/Reaxys/Reaxys_biocatalysis |
Top-N result to add to graph | 6 |
Feasible Synthetic Routes
Q1: How does 2-fluoro-3'-deoxyadenosine exert its trypanocidal activity, and how does its structure relate to this effect?
A1: this compound (also known as 2-fluorocordycepin) exhibits its trypanocidal activity by targeting the parasite Trypanosoma brucei through a multi-step process []:
- Uptake: The compound utilizes the parasite's high-affinity TbAT1/P2 adenosine transporter to enter the cell []. This transporter is essential for adenosine uptake in T. brucei, making it a potential target for therapeutic intervention.
- Phosphorylation: Once inside the parasite, this compound is phosphorylated by T. b. brucei adenosine kinase []. This phosphorylation step is crucial for the compound's activity, as it traps it within the parasite and allows it to interfere with essential metabolic processes.
- Mechanism of Action: Although the exact mechanism of action remains to be fully elucidated, it is believed that phosphorylated this compound interferes with nucleic acid synthesis, ultimately leading to parasite death [].
- 3'-Deoxyadenosine backbone: This structure mimics adenosine, allowing the compound to utilize the parasite's adenosine transporter for cellular entry [].
- 2-Fluoro substitution: This modification confers resistance to adenosine deaminase (ADA) [], an enzyme that rapidly degrades the parent compound cordycepin (3'-deoxyadenosine) in vivo. This resistance is crucial for its efficacy in animal models, as it allows for sustained therapeutic levels of the compound.
Q2: What are the preclinical findings regarding this compound's potential as a treatment for African trypanosomiasis?
A2: Preclinical studies have shown promising results regarding this compound as a potential therapeutic agent for African trypanosomiasis []:
- In vitro efficacy: The compound demonstrates potent trypanocidal activity against T. brucei in vitro [], highlighting its potential as a therapeutic agent.
- In vivo efficacy: Notably, this compound successfully cured T. brucei infection in a mouse model [], demonstrating its ability to effectively target the parasite in vivo.
Disclaimer and Information on In-Vitro Research Products
Please be aware that all articles and product information presented on BenchChem are intended solely for informational purposes. The products available for purchase on BenchChem are specifically designed for in-vitro studies, which are conducted outside of living organisms. In-vitro studies, derived from the Latin term "in glass," involve experiments performed in controlled laboratory settings using cells or tissues. It is important to note that these products are not categorized as medicines or drugs, and they have not received approval from the FDA for the prevention, treatment, or cure of any medical condition, ailment, or disease. We must emphasize that any form of bodily introduction of these products into humans or animals is strictly prohibited by law. It is essential to adhere to these guidelines to ensure compliance with legal and ethical standards in research and experimentation.