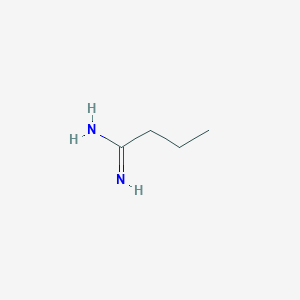
Butanimidamide
Overview
Description
Preparation Methods
Synthetic Routes and Reaction Conditions
Butanimidamide can be synthesized through several methods. One common approach involves the reaction of butanoic acid with ammonia, followed by the addition of a dehydrating agent to form butyramide. Another method involves the reaction of butanoic acid chloride with ammonia or an amine to yield butyramide.
Industrial Production Methods
In an industrial setting, this compound is typically produced through the catalytic hydrogenation of butyronitrile. This process involves the use of a metal catalyst, such as palladium or platinum, under high pressure and temperature conditions. The reaction proceeds as follows:
C4H7CN+2H2→C4H9NH2
Chemical Reactions Analysis
Types of Reactions
Butanimidamide undergoes various chemical reactions, including:
Oxidation: this compound can be oxidized to form butanoic acid.
Reduction: It can be reduced to form butylamine.
Substitution: It can undergo nucleophilic substitution reactions, where the amidine group is replaced by other functional groups.
Common Reagents and Conditions
Oxidation: Common oxidizing agents include potassium permanganate (KMnO4) and chromium trioxide (CrO3).
Reduction: Reducing agents such as lithium aluminum hydride (LiAlH4) or sodium borohydride (NaBH4) are commonly used.
Substitution: Nucleophiles such as hydroxide ions (OH-) or alkoxide ions (RO-) can be used in substitution reactions.
Major Products Formed
Oxidation: Butanoic acid
Reduction: Butylamine
Substitution: Various substituted amidines, depending on the nucleophile used.
Scientific Research Applications
Butanimidamide has several applications in scientific research:
Chemistry: It is used as a building block in the synthesis of more complex organic compounds.
Biology: It is used in the study of enzyme-substrate interactions and protein-ligand binding.
Industry: It is used in the production of polymers and other industrial chemicals.
Mechanism of Action
The mechanism of action of butanimidamide involves its interaction with specific molecular targets, such as enzymes or receptors. The amidine group can form hydrogen bonds and electrostatic interactions with these targets, leading to changes in their activity or function. This can result in various biological effects, depending on the specific target and pathway involved.
Comparison with Similar Compounds
Butanimidamide is similar to other amidine compounds, such as acetamidamide and propionamidamide. it is unique in its specific structure and reactivity. Compared to acetamidamide, this compound has a longer carbon chain, which can influence its physical and chemical properties. Similarly, compared to propionamidamide, this compound has different reactivity due to the presence of an additional carbon atom.
List of Similar Compounds
- Acetamidamide
- Propionamidamide
- Benzamidamide
Properties
IUPAC Name |
butanimidamide | |
---|---|---|
Source | PubChem | |
URL | https://pubchem.ncbi.nlm.nih.gov | |
Description | Data deposited in or computed by PubChem | |
InChI |
InChI=1S/C4H10N2/c1-2-3-4(5)6/h2-3H2,1H3,(H3,5,6) | |
Source | PubChem | |
URL | https://pubchem.ncbi.nlm.nih.gov | |
Description | Data deposited in or computed by PubChem | |
InChI Key |
RXKUYBRRTKRGME-UHFFFAOYSA-N | |
Source | PubChem | |
URL | https://pubchem.ncbi.nlm.nih.gov | |
Description | Data deposited in or computed by PubChem | |
Canonical SMILES |
CCCC(=N)N | |
Source | PubChem | |
URL | https://pubchem.ncbi.nlm.nih.gov | |
Description | Data deposited in or computed by PubChem | |
Molecular Formula |
C4H10N2 | |
Source | PubChem | |
URL | https://pubchem.ncbi.nlm.nih.gov | |
Description | Data deposited in or computed by PubChem | |
DSSTOX Substance ID |
DTXSID60274604 | |
Record name | Butanimidamide | |
Source | EPA DSSTox | |
URL | https://comptox.epa.gov/dashboard/DTXSID60274604 | |
Description | DSSTox provides a high quality public chemistry resource for supporting improved predictive toxicology. | |
Molecular Weight |
86.14 g/mol | |
Source | PubChem | |
URL | https://pubchem.ncbi.nlm.nih.gov | |
Description | Data deposited in or computed by PubChem | |
CAS No. |
107-90-4 | |
Record name | Butanimidamide | |
Source | CAS Common Chemistry | |
URL | https://commonchemistry.cas.org/detail?cas_rn=107-90-4 | |
Description | CAS Common Chemistry is an open community resource for accessing chemical information. Nearly 500,000 chemical substances from CAS REGISTRY cover areas of community interest, including common and frequently regulated chemicals, and those relevant to high school and undergraduate chemistry classes. This chemical information, curated by our expert scientists, is provided in alignment with our mission as a division of the American Chemical Society. | |
Explanation | The data from CAS Common Chemistry is provided under a CC-BY-NC 4.0 license, unless otherwise stated. | |
Record name | Butanimidamide | |
Source | DTP/NCI | |
URL | https://dtp.cancer.gov/dtpstandard/servlet/dwindex?searchtype=NSC&outputformat=html&searchlist=56360 | |
Description | The NCI Development Therapeutics Program (DTP) provides services and resources to the academic and private-sector research communities worldwide to facilitate the discovery and development of new cancer therapeutic agents. | |
Explanation | Unless otherwise indicated, all text within NCI products is free of copyright and may be reused without our permission. Credit the National Cancer Institute as the source. | |
Record name | Butanimidamide | |
Source | EPA DSSTox | |
URL | https://comptox.epa.gov/dashboard/DTXSID60274604 | |
Description | DSSTox provides a high quality public chemistry resource for supporting improved predictive toxicology. | |
Record name | BUTYRAMIDINE | |
Source | FDA Global Substance Registration System (GSRS) | |
URL | https://gsrs.ncats.nih.gov/ginas/app/beta/substances/HSB7V5A6Z6 | |
Description | The FDA Global Substance Registration System (GSRS) enables the efficient and accurate exchange of information on what substances are in regulated products. Instead of relying on names, which vary across regulatory domains, countries, and regions, the GSRS knowledge base makes it possible for substances to be defined by standardized, scientific descriptions. | |
Explanation | Unless otherwise noted, the contents of the FDA website (www.fda.gov), both text and graphics, are not copyrighted. They are in the public domain and may be republished, reprinted and otherwise used freely by anyone without the need to obtain permission from FDA. Credit to the U.S. Food and Drug Administration as the source is appreciated but not required. | |
Retrosynthesis Analysis
AI-Powered Synthesis Planning: Our tool employs the Template_relevance Pistachio, Template_relevance Bkms_metabolic, Template_relevance Pistachio_ringbreaker, Template_relevance Reaxys, Template_relevance Reaxys_biocatalysis model, leveraging a vast database of chemical reactions to predict feasible synthetic routes.
One-Step Synthesis Focus: Specifically designed for one-step synthesis, it provides concise and direct routes for your target compounds, streamlining the synthesis process.
Accurate Predictions: Utilizing the extensive PISTACHIO, BKMS_METABOLIC, PISTACHIO_RINGBREAKER, REAXYS, REAXYS_BIOCATALYSIS database, our tool offers high-accuracy predictions, reflecting the latest in chemical research and data.
Strategy Settings
Precursor scoring | Relevance Heuristic |
---|---|
Min. plausibility | 0.01 |
Model | Template_relevance |
Template Set | Pistachio/Bkms_metabolic/Pistachio_ringbreaker/Reaxys/Reaxys_biocatalysis |
Top-N result to add to graph | 6 |
Feasible Synthetic Routes
Disclaimer and Information on In-Vitro Research Products
Please be aware that all articles and product information presented on BenchChem are intended solely for informational purposes. The products available for purchase on BenchChem are specifically designed for in-vitro studies, which are conducted outside of living organisms. In-vitro studies, derived from the Latin term "in glass," involve experiments performed in controlled laboratory settings using cells or tissues. It is important to note that these products are not categorized as medicines or drugs, and they have not received approval from the FDA for the prevention, treatment, or cure of any medical condition, ailment, or disease. We must emphasize that any form of bodily introduction of these products into humans or animals is strictly prohibited by law. It is essential to adhere to these guidelines to ensure compliance with legal and ethical standards in research and experimentation.