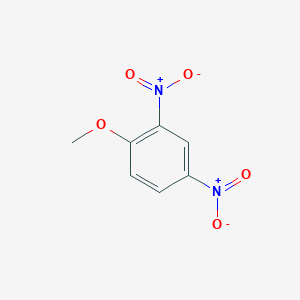
2,4-Dinitroanisole
Overview
Description
2,4-Dinitroanisole is an organic compound with the chemical formula C7H6N2O5. It is characterized by an anisole (methoxybenzene) core with two nitro groups (–NO2) attached at the 2 and 4 positions. This compound is known for its pale yellow granular crystals or needles and has a melting point of 94.5°C . Historically, this compound has been used as an explosive in warheads and is currently being investigated as a replacement for 2,4,6-Trinitrotoluene in melt-cast insensitive munitions .
Mechanism of Action
Target of Action
2,4-Dinitroanisole (DNAN) is a low sensitivity organic compound . It has an anisole (methoxybenzene) core, with two nitro groups (–NO2) attached .
Mode of Action
The mode of action of DNAN involves several chemical reactions. For instance, DNAN reacts without heat with a potassium cyanide solution to form a red-colored product via the isopurpuric acid reaction . In this reaction, cyanide is added in the meta position, and the ortho nitro group is reduced to -NHOH .
Biochemical Pathways
The biochemical pathways of DNAN involve various transformations. For example, in alkaline conditions, DNAN can be attacked at the methoxy position with nucleophiles to form Meisenheimer complexes . In these complexes, the ring develops a negative charge and another group is attached at the methoxy attachment point . Furthermore, when heated under pressure with water and ammonia, DNAN is converted to 2,4-dinitroaniline .
Pharmacokinetics
It is known that dnan is metabolized in vivo to 2,4-dinitrophenol (dnp), but other details of its metabolism or pharmacokinetics are unknown .
Result of Action
The result of DNAN’s action depends on the specific context. For instance, in the context of explosives, DNAN is being tested by the Military Industry as a replacement for 2,4,6-trinitrotoluene (TNT) in explosive formulations . It is a less sensitive explosive and can be handled and transported with less stringent conditions .
Action Environment
The action of DNAN can be influenced by various environmental factors. For instance, DNAN is a stable compound and has a much higher melting point compared to TNT . It is soluble in ethanol, ether, and benzene, and very slightly soluble in water . The initial decomposition temperature is 295 °C, and the explosion temperature is 312 °C . On explosion, the adiabatic temperature rise is 4923 °C . Therefore, factors such as temperature, solvent, and pressure can significantly influence the action, efficacy, and stability of DNAN.
Biochemical Analysis
Biochemical Properties
The transformation involves the formation of 2-amino-4-nitroanisole as the major end-product via the intermediary formation of the arylnitroso (ArNO) and arylhydroxylamino (ArNHOH) derivatives .
Molecular Mechanism
The molecular mechanism of 2,4-Dinitroanisole involves the reduction of the ortho-nitro group to form 2-amino-4-nitroanisole . This reaction is facilitated by the arylnitroso (ArNO) and arylhydroxylamino (ArNHOH) derivatives .
Temporal Effects in Laboratory Settings
In laboratory settings, this compound was completely transformed in 8 days in soil slurries supplemented with carbon and nitrogen sources . It was completely transformed in 34 days in slurries supplemented with carbons alone and persisted in unamended microcosms .
Metabolic Pathways
The metabolic pathways of this compound involve the reduction of the ortho-nitro group to form 2-amino-4-nitroanisole . This reaction is facilitated by the arylnitroso (ArNO) and arylhydroxylamino (ArNHOH) derivatives .
Preparation Methods
The synthesis of 2,4-Dinitroanisole has evolved over the years. It can be prepared by several methods starting with different raw materials, including chlorobenzene, phenol, 1-chloro-2,4-dinitrobenzene, and anisole . One common method involves the nitration of anisole using a mixture of propionic anhydride and 98% nitric acid to form the reactive nitrating species, proprionyl nitrate. This reagent is then added to a highly concentrated solution mixture of anisole and propionic anhydride to produce this compound . Industrial production methods have been optimized to use less harsh conditions and address environmental concerns .
Chemical Reactions Analysis
2,4-Dinitroanisole undergoes various chemical reactions, including:
Nucleophilic Substitution: In alkaline conditions, it can be attacked at the methoxy position with nucleophiles to form Meisenheimer complexes.
Hydrolysis: When heated under pressure with water and ammonia, it is converted to 2,4-dinitroaniline.
Common reagents used in these reactions include potassium cyanide, sodium methoxide, and ammonia. Major products formed include 2,4-dinitroaniline and various Meisenheimer complexes .
Scientific Research Applications
2,4-Dinitroanisole has diverse applications in scientific research:
Comparison with Similar Compounds
2,4-Dinitroanisole is often compared with other nitroaromatic compounds such as 2,4,6-Trinitrotoluene and 2,6-Dinitroanisole. Compared to 2,4,6-Trinitrotoluene, this compound has a higher melting point, is less dense, and has only 90% of the explosive power . It is also less toxic and cheaper to manufacture . The unique properties of this compound, such as its reduced sensitivity and higher thermal stability, make it a promising alternative for various applications .
Similar compounds include:
- 2,4,6-Trinitrotoluene
- 2,6-Dinitroanisole
- 2,4-Dinitroaniline
Properties
IUPAC Name |
1-methoxy-2,4-dinitrobenzene | |
---|---|---|
Source | PubChem | |
URL | https://pubchem.ncbi.nlm.nih.gov | |
Description | Data deposited in or computed by PubChem | |
InChI |
InChI=1S/C7H6N2O5/c1-14-7-3-2-5(8(10)11)4-6(7)9(12)13/h2-4H,1H3 | |
Source | PubChem | |
URL | https://pubchem.ncbi.nlm.nih.gov | |
Description | Data deposited in or computed by PubChem | |
InChI Key |
CVYZVNVPQRKDLW-UHFFFAOYSA-N | |
Source | PubChem | |
URL | https://pubchem.ncbi.nlm.nih.gov | |
Description | Data deposited in or computed by PubChem | |
Canonical SMILES |
COC1=C(C=C(C=C1)[N+](=O)[O-])[N+](=O)[O-] | |
Source | PubChem | |
URL | https://pubchem.ncbi.nlm.nih.gov | |
Description | Data deposited in or computed by PubChem | |
Molecular Formula |
C7H6N2O5 | |
Source | PubChem | |
URL | https://pubchem.ncbi.nlm.nih.gov | |
Description | Data deposited in or computed by PubChem | |
DSSTOX Substance ID |
DTXSID9041366 | |
Record name | 2,4-Dinitroanisole | |
Source | EPA DSSTox | |
URL | https://comptox.epa.gov/dashboard/DTXSID9041366 | |
Description | DSSTox provides a high quality public chemistry resource for supporting improved predictive toxicology. | |
Molecular Weight |
198.13 g/mol | |
Source | PubChem | |
URL | https://pubchem.ncbi.nlm.nih.gov | |
Description | Data deposited in or computed by PubChem | |
Physical Description |
Dry Powder; Other Solid, Tan powder; [Sigma-Aldrich MSDS] | |
Record name | Benzene, 1-methoxy-2,4-dinitro- | |
Source | EPA Chemicals under the TSCA | |
URL | https://www.epa.gov/chemicals-under-tsca | |
Description | EPA Chemicals under the Toxic Substances Control Act (TSCA) collection contains information on chemicals and their regulations under TSCA, including non-confidential content from the TSCA Chemical Substance Inventory and Chemical Data Reporting. | |
Record name | 2,4-Dinitroanisole | |
Source | Haz-Map, Information on Hazardous Chemicals and Occupational Diseases | |
URL | https://haz-map.com/Agents/19472 | |
Description | Haz-Map® is an occupational health database designed for health and safety professionals and for consumers seeking information about the adverse effects of workplace exposures to chemical and biological agents. | |
Explanation | Copyright (c) 2022 Haz-Map(R). All rights reserved. Unless otherwise indicated, all materials from Haz-Map are copyrighted by Haz-Map(R). No part of these materials, either text or image may be used for any purpose other than for personal use. Therefore, reproduction, modification, storage in a retrieval system or retransmission, in any form or by any means, electronic, mechanical or otherwise, for reasons other than personal use, is strictly prohibited without prior written permission. | |
Vapor Pressure |
0.000138 [mmHg] | |
Record name | 2,4-Dinitroanisole | |
Source | Haz-Map, Information on Hazardous Chemicals and Occupational Diseases | |
URL | https://haz-map.com/Agents/19472 | |
Description | Haz-Map® is an occupational health database designed for health and safety professionals and for consumers seeking information about the adverse effects of workplace exposures to chemical and biological agents. | |
Explanation | Copyright (c) 2022 Haz-Map(R). All rights reserved. Unless otherwise indicated, all materials from Haz-Map are copyrighted by Haz-Map(R). No part of these materials, either text or image may be used for any purpose other than for personal use. Therefore, reproduction, modification, storage in a retrieval system or retransmission, in any form or by any means, electronic, mechanical or otherwise, for reasons other than personal use, is strictly prohibited without prior written permission. | |
CAS No. |
119-27-7 | |
Record name | 2,4-Dinitroanisole | |
Source | CAS Common Chemistry | |
URL | https://commonchemistry.cas.org/detail?cas_rn=119-27-7 | |
Description | CAS Common Chemistry is an open community resource for accessing chemical information. Nearly 500,000 chemical substances from CAS REGISTRY cover areas of community interest, including common and frequently regulated chemicals, and those relevant to high school and undergraduate chemistry classes. This chemical information, curated by our expert scientists, is provided in alignment with our mission as a division of the American Chemical Society. | |
Explanation | The data from CAS Common Chemistry is provided under a CC-BY-NC 4.0 license, unless otherwise stated. | |
Record name | 2,4-Dinitroanisole | |
Source | ChemIDplus | |
URL | https://pubchem.ncbi.nlm.nih.gov/substance/?source=chemidplus&sourceid=0000119277 | |
Description | ChemIDplus is a free, web search system that provides access to the structure and nomenclature authority files used for the identification of chemical substances cited in National Library of Medicine (NLM) databases, including the TOXNET system. | |
Record name | 2,4-DINITROANISOLE | |
Source | DTP/NCI | |
URL | https://dtp.cancer.gov/dtpstandard/servlet/dwindex?searchtype=NSC&outputformat=html&searchlist=8733 | |
Description | The NCI Development Therapeutics Program (DTP) provides services and resources to the academic and private-sector research communities worldwide to facilitate the discovery and development of new cancer therapeutic agents. | |
Explanation | Unless otherwise indicated, all text within NCI products is free of copyright and may be reused without our permission. Credit the National Cancer Institute as the source. | |
Record name | Benzene, 1-methoxy-2,4-dinitro- | |
Source | EPA Chemicals under the TSCA | |
URL | https://www.epa.gov/chemicals-under-tsca | |
Description | EPA Chemicals under the Toxic Substances Control Act (TSCA) collection contains information on chemicals and their regulations under TSCA, including non-confidential content from the TSCA Chemical Substance Inventory and Chemical Data Reporting. | |
Record name | 2,4-Dinitroanisole | |
Source | EPA DSSTox | |
URL | https://comptox.epa.gov/dashboard/DTXSID9041366 | |
Description | DSSTox provides a high quality public chemistry resource for supporting improved predictive toxicology. | |
Record name | 2,4-dinitroanisole | |
Source | European Chemicals Agency (ECHA) | |
URL | https://echa.europa.eu/substance-information/-/substanceinfo/100.003.919 | |
Description | The European Chemicals Agency (ECHA) is an agency of the European Union which is the driving force among regulatory authorities in implementing the EU's groundbreaking chemicals legislation for the benefit of human health and the environment as well as for innovation and competitiveness. | |
Explanation | Use of the information, documents and data from the ECHA website is subject to the terms and conditions of this Legal Notice, and subject to other binding limitations provided for under applicable law, the information, documents and data made available on the ECHA website may be reproduced, distributed and/or used, totally or in part, for non-commercial purposes provided that ECHA is acknowledged as the source: "Source: European Chemicals Agency, http://echa.europa.eu/". Such acknowledgement must be included in each copy of the material. ECHA permits and encourages organisations and individuals to create links to the ECHA website under the following cumulative conditions: Links can only be made to webpages that provide a link to the Legal Notice page. | |
Record name | 2,4-DINITROANISOLE | |
Source | FDA Global Substance Registration System (GSRS) | |
URL | https://gsrs.ncats.nih.gov/ginas/app/beta/substances/1L0OD70295 | |
Description | The FDA Global Substance Registration System (GSRS) enables the efficient and accurate exchange of information on what substances are in regulated products. Instead of relying on names, which vary across regulatory domains, countries, and regions, the GSRS knowledge base makes it possible for substances to be defined by standardized, scientific descriptions. | |
Explanation | Unless otherwise noted, the contents of the FDA website (www.fda.gov), both text and graphics, are not copyrighted. They are in the public domain and may be republished, reprinted and otherwise used freely by anyone without the need to obtain permission from FDA. Credit to the U.S. Food and Drug Administration as the source is appreciated but not required. | |
Synthesis routes and methods
Procedure details
Retrosynthesis Analysis
AI-Powered Synthesis Planning: Our tool employs the Template_relevance Pistachio, Template_relevance Bkms_metabolic, Template_relevance Pistachio_ringbreaker, Template_relevance Reaxys, Template_relevance Reaxys_biocatalysis model, leveraging a vast database of chemical reactions to predict feasible synthetic routes.
One-Step Synthesis Focus: Specifically designed for one-step synthesis, it provides concise and direct routes for your target compounds, streamlining the synthesis process.
Accurate Predictions: Utilizing the extensive PISTACHIO, BKMS_METABOLIC, PISTACHIO_RINGBREAKER, REAXYS, REAXYS_BIOCATALYSIS database, our tool offers high-accuracy predictions, reflecting the latest in chemical research and data.
Strategy Settings
Precursor scoring | Relevance Heuristic |
---|---|
Min. plausibility | 0.01 |
Model | Template_relevance |
Template Set | Pistachio/Bkms_metabolic/Pistachio_ringbreaker/Reaxys/Reaxys_biocatalysis |
Top-N result to add to graph | 6 |
Feasible Synthetic Routes
Q1: What are the key structural characteristics of 2,4-Dinitroanisole?
A1: this compound has the molecular formula C7H6N2O5 and a molecular weight of 198.14 g/mol []. Its structure consists of a benzene ring with a methoxy group (–OCH3) and two nitro groups (–NO2) attached to it []. Spectroscopic data, including FTIR and NMR, have been used to confirm its structure [, ].
Q2: Why is this compound being considered as a replacement for 2,4,6-trinitrotoluene (TNT)?
A2: DNAN is being explored as a less sensitive alternative to TNT in melt-cast explosive formulations [, , , ]. It exhibits a reduced sensitivity to accidental detonation while offering comparable explosive properties, making it a safer option for handling and transport [, ].
Q3: Are there any challenges associated with using this compound in melt-cast formulations?
A3: Yes, one challenge is the irreversible growth observed in DNAN-based formulations during heat cycling, which can reach up to 15% volume increase []. This has prompted investigations into the polymorphism of DNAN to understand and potentially mitigate this issue [].
Q4: How does the particle size of other explosive components affect DNAN-based formulations?
A4: Research suggests that smaller particle sizes of granular explosive components, like HMX, within aluminized DNAN-based melt-cast explosives lead to faster detonation growth and shock wave propagation []. This highlights the importance of particle size control in optimizing the performance of these formulations.
Q5: Has the crystal structure of this compound been studied and what does it reveal?
A5: Yes, studies have revealed that this compound exists in two polymorphic forms at ambient conditions: the thermodynamically stable α-form and the kinetic β-form [, , ]. Notably, the oxygen atoms of the nitro groups in both forms exhibit disorder over two positions at room temperature [, ].
Q6: How does temperature affect the polymorphic forms of DNAN?
A6: The β-form undergoes a low-temperature phase transition at 264.5 K []. This transition leads to a significant change in the β angle of the unit cell and results in an unusual biaxial negative thermal expansion along the b-axis [].
Q7: Can the crystal morphology and polymorphism of this compound be controlled?
A7: Research suggests that factors like solvent, solution concentration, and the presence of additives can influence the morphology and polymorphism of DNAN crystals []. Certain additives, particularly those containing aromatic or nitro groups, might compete with DNAN's intermolecular interactions, thereby affecting crystal growth and polymorphism.
Q8: Are there enzymatic pathways involved in this compound degradation?
A8: Yes, bacteria like Nocardioides sp. JS1661 have demonstrated the ability to degrade DNAN via enzymatic hydrolysis [, ]. This process involves the hydrolytic release of methanol, forming 2,4-dinitrophenol (DNP), which is further degraded by a pathway involving a hydride-Meisenheimer complex and nitrite release [].
Q9: How do the mechanisms of alkaline and enzymatic hydrolysis of DNAN compare?
A9: While both processes result in 2,4-dinitrophenol (DNP) as the final product, they proceed through different mechanisms, as evidenced by distinct carbon and nitrogen isotope fractionation patterns []. This highlights the diverse ways in which DNAN can be transformed in the environment.
Q10: Can compound specific isotope analysis (CSIA) be used to monitor DNAN degradation?
A10: Yes, CSIA, particularly using carbon and nitrogen isotopes, has proven valuable in assessing the extent and pathways of DNAN transformation in various environmental matrices [, , , ]. Each degradation pathway, whether abiotic or biotic, tends to impart a characteristic isotopic signature on the remaining DNAN and its degradation products, enabling researchers to differentiate between them.
Q11: What are the environmental concerns associated with this compound?
A11: Being an insensitive munition compound, DNAN poses a potential contamination risk to soil and water systems [, , ]. Its degradation products, such as 2,4-dinitrophenol and various aromatic amines, can be toxic and persistent in the environment, necessitating a thorough understanding of its fate and transport [, , , ].
Q12: How is this compound biotransformed in the environment?
A12: Studies using anaerobic sludge have shown that DNAN is primarily biotransformed through the reductive transformation of its nitro groups to amino groups, forming intermediates like 2-methoxy-5-nitroaniline and ultimately leading to 2,4-diaminoanisole [, ]. This process is influenced by factors like temperature, electron donor availability, and microbial community composition [, ].
Q13: What is known about the toxicity of this compound in animals?
A13: Studies in rats have identified several toxicity concerns. DNAN appears to be metabolized to 2,4-dinitrophenol, which disrupts energy homeostasis and potentially leads to reduced feed efficiency and weight loss, particularly in males [, , ].
Q14: Are there specific organs targeted by DNAN toxicity?
A14: Yes, DNAN exhibits toxicity towards specific organs in rats. These include:
- Blood: DNAN induces anemia, splenic enlargement, hemosiderosis, and extramedullary hematopoiesis, with females showing greater sensitivity [, , ].
- Testes: It causes testicular atrophy, degeneration of seminiferous tubules, and reduced sperm production [, ].
- Nervous System: DNAN exposure can lead to gait abnormalities, stereotypical behavior, and cerebellar lesions, suggesting neurotoxicity [, ].
Q15: How is this compound absorbed and metabolized in mammals?
A15: Studies in rhesus macaques indicate that DNAN is readily absorbed after oral ingestion and metabolized to 2,4-dinitrophenol (DNP) []. Notably, blood DNP concentrations were observed to be significantly higher than the parent compound, highlighting the importance of monitoring DNP levels as a biomarker of DNAN exposure [, ].
Q16: What is the recommended workplace exposure limit for DNAN?
A16: Based on toxicity data from both DNAN and its metabolite, DNP, the recommended workplace environmental exposure limit for DNAN is 0.1 mg/m3, time-weighted average for an 8-hour workday [].
Q17: What analytical methods are employed for the detection and quantification of this compound?
A17: Several analytical techniques have been developed and utilized for DNAN analysis, including:
- **High-performance liquid chromatography (HPLC) ** [, , ]
- **Gas chromatography-mass spectrometry (GC-MS) ** [, ]
- **Ultrafast liquid chromatography-tandem mass spectrometry (UFLC-MS/MS) ** []
- **Ion chromatography (IC) ** []
Q18: Have any remediation strategies for DNAN contamination been explored?
A18: Research suggests several promising remediation approaches:
- Anaerobic bioreactors: Fluidized bed bioreactors, using electron donors like ethanol, have demonstrated effective removal of DNAN from simulated wastewater [, , ].
- Bioaugmentation with specific bacterial strains: Introducing bacterial species capable of degrading DNAN, such as Nocardioides sp. JS1661 or Geobacter metallireducens, shows potential for enhancing bioremediation [, , ].
- Phytoremediation: Preliminary research suggests that plants like Arabidopsis thaliana can take up, metabolize, and potentially remediate DNAN from contaminated water [].
Disclaimer and Information on In-Vitro Research Products
Please be aware that all articles and product information presented on BenchChem are intended solely for informational purposes. The products available for purchase on BenchChem are specifically designed for in-vitro studies, which are conducted outside of living organisms. In-vitro studies, derived from the Latin term "in glass," involve experiments performed in controlled laboratory settings using cells or tissues. It is important to note that these products are not categorized as medicines or drugs, and they have not received approval from the FDA for the prevention, treatment, or cure of any medical condition, ailment, or disease. We must emphasize that any form of bodily introduction of these products into humans or animals is strictly prohibited by law. It is essential to adhere to these guidelines to ensure compliance with legal and ethical standards in research and experimentation.