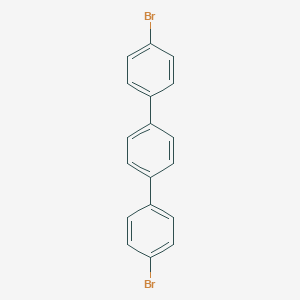
4,4''-Dibromo-p-terphenyl
Overview
Description
4,4’‘-Dibromo-p-terphenyl is an organic compound with the molecular formula C18H12Br2. It is a derivative of p-terphenyl, where two bromine atoms are substituted at the 4 and 4’’ positions of the terphenyl structure. This compound is known for its applications in organic synthesis, particularly in the preparation of liquid crystals and organic light-emitting diodes (OLEDs) due to its unique electronic properties .
Scientific Research Applications
4,4’'-Dibromo-p-terphenyl has several applications in scientific research:
Organic Synthesis: It is used as a building block for the synthesis of complex organic molecules.
Liquid Crystals: It is a precursor for the synthesis of liquid crystal compounds used in display technologies.
Organic Light-Emitting Diodes (OLEDs): It is used in the development of OLED materials due to its favorable electronic properties.
Nanotechnology: It is employed in the fabrication of graphene nanoribbons and other nanostructures.
Mechanism of Action
Target of Action
4,4’'-Dibromo-p-terphenyl (DBTP) is primarily used as a key intermediate in the synthesis of terphenyl derivatives, liquid crystals, and certain organic electroluminescent materials . The primary targets of DBTP are therefore the molecular structures that these materials form.
Mode of Action
DBTP undergoes a process known as Ullmann coupling when thermally catalyzed on a surface such as Ag(111). This reaction involves the formation of carbon-carbon bonds at a single molecular level . The DBTP molecules partially debrominate and align along the surface after deposition at room temperature .
Biochemical Pathways
These activities include strong immunosuppressive activity, neuroprotective effects, coagulation effects, thrombosis, isomeric 5-lipoxygenase inhibitory activity, and cytotoxic activity .
Result of Action
The primary result of DBTP’s action is the formation of new molecular structures through the Ullmann coupling reaction. This reaction results in the formation of carbon-carbon bonds, leading to the creation of terphenyl derivatives, liquid crystals, and organic electroluminescent materials .
Safety and Hazards
Safety data sheets indicate that 4,4’'-Dibromo-p-terphenyl is considered hazardous by the 2012 OSHA Hazard Communication Standard . It is recommended to avoid breathing mist, gas, or vapors, avoid contact with skin and eyes, use personal protective equipment, wear chemical impermeable gloves, ensure adequate ventilation, remove all sources of ignition, evacuate personnel to safe areas, and keep people away from and upwind of spill/leak .
Future Directions
Preparation Methods
Synthetic Routes and Reaction Conditions
4,4’'-Dibromo-p-terphenyl can be synthesized through the bromination of p-terphenyl. The typical synthetic route involves the following steps :
Starting Materials:
p-terphenyl, liquid bromine, and an organic solvent such as acetonitrile.Reaction Conditions: The reaction is carried out at room temperature with the addition of iron powder as a catalyst.
Industrial Production Methods
In industrial settings, the synthesis of 4,4’'-Dibromo-p-terphenyl follows similar principles but on a larger scale. The use of continuous flow reactors and automated systems ensures higher efficiency and yield .
Chemical Reactions Analysis
Types of Reactions
4,4’'-Dibromo-p-terphenyl undergoes various chemical reactions, including:
Substitution Reactions: The bromine atoms can be substituted with other functional groups through nucleophilic substitution reactions.
Coupling Reactions: It participates in Ullmann coupling reactions to form larger aromatic systems.
Common Reagents and Conditions
Nucleophilic Substitution: Common reagents include sodium methoxide, potassium tert-butoxide, and other strong nucleophiles.
Ullmann Coupling: Typically involves the use of copper catalysts and elevated temperatures.
Major Products
Substitution Products: Depending on the nucleophile used, various substituted terphenyl derivatives can be obtained.
Coupling Products: Larger aromatic systems and polymers can be synthesized through Ullmann coupling.
Comparison with Similar Compounds
Similar Compounds
4,4’'-Dibromobiphenyl: Similar in structure but with only two phenyl rings.
1,3,5-Tris(4-bromophenyl)benzene: Contains three brominated phenyl rings attached to a central benzene ring.
4,4’'-Diiodobiphenyl: Similar structure with iodine atoms instead of bromine.
Uniqueness
4,4’'-Dibromo-p-terphenyl is unique due to its three phenyl rings and the specific positioning of bromine atoms, which confer distinct electronic properties. This makes it particularly useful in the synthesis of liquid crystals and OLED materials, where precise electronic characteristics are essential .
Properties
IUPAC Name |
1,4-bis(4-bromophenyl)benzene | |
---|---|---|
Source | PubChem | |
URL | https://pubchem.ncbi.nlm.nih.gov | |
Description | Data deposited in or computed by PubChem | |
InChI |
InChI=1S/C18H12Br2/c19-17-9-5-15(6-10-17)13-1-2-14(4-3-13)16-7-11-18(20)12-8-16/h1-12H | |
Source | PubChem | |
URL | https://pubchem.ncbi.nlm.nih.gov | |
Description | Data deposited in or computed by PubChem | |
InChI Key |
VAIPJQIPFPRJKJ-UHFFFAOYSA-N | |
Source | PubChem | |
URL | https://pubchem.ncbi.nlm.nih.gov | |
Description | Data deposited in or computed by PubChem | |
Canonical SMILES |
C1=CC(=CC=C1C2=CC=C(C=C2)Br)C3=CC=C(C=C3)Br | |
Source | PubChem | |
URL | https://pubchem.ncbi.nlm.nih.gov | |
Description | Data deposited in or computed by PubChem | |
Molecular Formula |
C18H12Br2 | |
Source | PubChem | |
URL | https://pubchem.ncbi.nlm.nih.gov | |
Description | Data deposited in or computed by PubChem | |
DSSTOX Substance ID |
DTXSID40498987 | |
Record name | 1~4~,3~4~-Dibromo-1~1~,2~1~:2~4~,3~1~-terphenyl | |
Source | EPA DSSTox | |
URL | https://comptox.epa.gov/dashboard/DTXSID40498987 | |
Description | DSSTox provides a high quality public chemistry resource for supporting improved predictive toxicology. | |
Molecular Weight |
388.1 g/mol | |
Source | PubChem | |
URL | https://pubchem.ncbi.nlm.nih.gov | |
Description | Data deposited in or computed by PubChem | |
CAS No. |
17788-94-2 | |
Record name | 1~4~,3~4~-Dibromo-1~1~,2~1~:2~4~,3~1~-terphenyl | |
Source | EPA DSSTox | |
URL | https://comptox.epa.gov/dashboard/DTXSID40498987 | |
Description | DSSTox provides a high quality public chemistry resource for supporting improved predictive toxicology. | |
Record name | 4,4''-Dibromo-p-terphenyl | |
Source | European Chemicals Agency (ECHA) | |
URL | https://echa.europa.eu/information-on-chemicals | |
Description | The European Chemicals Agency (ECHA) is an agency of the European Union which is the driving force among regulatory authorities in implementing the EU's groundbreaking chemicals legislation for the benefit of human health and the environment as well as for innovation and competitiveness. | |
Explanation | Use of the information, documents and data from the ECHA website is subject to the terms and conditions of this Legal Notice, and subject to other binding limitations provided for under applicable law, the information, documents and data made available on the ECHA website may be reproduced, distributed and/or used, totally or in part, for non-commercial purposes provided that ECHA is acknowledged as the source: "Source: European Chemicals Agency, http://echa.europa.eu/". Such acknowledgement must be included in each copy of the material. ECHA permits and encourages organisations and individuals to create links to the ECHA website under the following cumulative conditions: Links can only be made to webpages that provide a link to the Legal Notice page. | |
Retrosynthesis Analysis
AI-Powered Synthesis Planning: Our tool employs the Template_relevance Pistachio, Template_relevance Bkms_metabolic, Template_relevance Pistachio_ringbreaker, Template_relevance Reaxys, Template_relevance Reaxys_biocatalysis model, leveraging a vast database of chemical reactions to predict feasible synthetic routes.
One-Step Synthesis Focus: Specifically designed for one-step synthesis, it provides concise and direct routes for your target compounds, streamlining the synthesis process.
Accurate Predictions: Utilizing the extensive PISTACHIO, BKMS_METABOLIC, PISTACHIO_RINGBREAKER, REAXYS, REAXYS_BIOCATALYSIS database, our tool offers high-accuracy predictions, reflecting the latest in chemical research and data.
Strategy Settings
Precursor scoring | Relevance Heuristic |
---|---|
Min. plausibility | 0.01 |
Model | Template_relevance |
Template Set | Pistachio/Bkms_metabolic/Pistachio_ringbreaker/Reaxys/Reaxys_biocatalysis |
Top-N result to add to graph | 6 |
Feasible Synthetic Routes
A: 4,4''-Dibromo-p-terphenyl (DBTP) readily adsorbs onto various metal surfaces, including gold (Au), silver (Ag), and copper (Cu). The bromine atoms in DBTP play a crucial role, undergoing catalytic cleavage (dehalogenation) on these surfaces. [, , , , , , , , , , , , , , ]. This dehalogenation leads to the formation of reactive radical species that can participate in various on-surface reactions, most notably Ullmann coupling [, , , , , , , ]. This process enables the formation of carbon-carbon bonds between adjacent DBTP molecules, ultimately leading to the creation of extended structures like poly(para-phenylene) (PPP) [, , , , , , , ]. Further processing can then transform these PPP chains into graphene nanoribbons (GNRs), highly sought-after materials for nanoelectronics [, , ].
A:
Molecular formula: C18H12Br2 []* Molecular weight: 388.09 g/mol * Spectroscopic data:* While the provided articles primarily focus on DBTP's on-surface reactivity and resulting structures, techniques like Raman spectroscopy are used to characterize the synthesized GNRs and confirm the absence of degradation products [].
A: The specific metal substrate significantly affects the reaction pathway and outcome. For instance, reversible dehalogenation is observed on Au(111), while on Cu(111), organometallic intermediates are predominantly formed, inhibiting reversibility []. Additionally, the activation temperatures for bromine scission and subsequent coupling reactions vary between different metals and their facets. For example, Au(110) and Au(100) require higher temperatures for Ullmann coupling and cyclodehydrogenation compared to Au(111) []. Ag(110) demonstrates intermediate properties, with the formation of organometallic intermediates involving silver adatoms before PPP formation [].
A: Yes, the presence of oxygen can significantly alter the reaction pathway of DBTP on metal surfaces. Instead of forming 1D polymers as seen in oxygen-free conditions, oxygen adsorption can promote the formation of 2D organometallic networks upon annealing []. This occurs due to oxygen stabilizing the organometallic segments formed during the reaction.
A: Density Functional Theory (DFT) calculations are extensively employed to complement experimental observations and provide insights into the energetics and mechanisms of DBTP's on-surface reactions [, , , , , ]. For example, DFT calculations revealed the energy gains associated with different configurations of DBTP molecules caged in porous supramolecular structures on Ag(111), stabilized by halogen and hydrogen bonds []. Additionally, DFT helps explain the different activation energies for bromine desorption from Ag and Au surfaces, explaining the varying kinetics of molecular chain and GNR formation [].
A: While the provided research focuses primarily on DBTP, studies using 1,4-dibromobenzene demonstrate that precursor length can influence the size of the resulting 2D organometallic networks on Cu(111) []. This suggests that structural modifications to the DBTP backbone could be used to tailor the properties and self-assembly of the resulting nanostructures.
A: Adding metal atom catalysts, such as cobalt, can significantly enhance the efficiency of the Ullmann coupling reaction involving DBTP on less reactive surfaces like TiO2(110) [, ]. Cobalt atoms facilitate debromination at room temperature, leading to a higher yield of poly-para-phenylene (PPP) by reducing the desorption of DBTP molecules during the reaction.
A: Yes, DBTP has been successfully used in conjunction with boron precursors to create mixed-dimensional heterostructures composed of 2D borophene and 1D armchair graphene nanoribbons (aGNRs) []. This self-assembly process highlights the versatility of DBTP as a building block for complex nanomaterials.
A: Utilizing light in conjunction with heat can significantly lower the activation temperatures for each stage of the Ullmann coupling reaction involving DBTP []. This light-assisted approach enhances the reactivity of less reactive surfaces, such as Ag(100), enabling PPP synthesis at lower temperatures and providing an additional parameter for controlling on-surface synthesis [].
A: Raman spectroscopy confirms the stability of GNRs synthesized from DBTP after exposure to air []. The presence of characteristic GNR vibrational modes and the absence of degradation-related fingerprints suggest their suitability for applications outside controlled environments.
Disclaimer and Information on In-Vitro Research Products
Please be aware that all articles and product information presented on BenchChem are intended solely for informational purposes. The products available for purchase on BenchChem are specifically designed for in-vitro studies, which are conducted outside of living organisms. In-vitro studies, derived from the Latin term "in glass," involve experiments performed in controlled laboratory settings using cells or tissues. It is important to note that these products are not categorized as medicines or drugs, and they have not received approval from the FDA for the prevention, treatment, or cure of any medical condition, ailment, or disease. We must emphasize that any form of bodily introduction of these products into humans or animals is strictly prohibited by law. It is essential to adhere to these guidelines to ensure compliance with legal and ethical standards in research and experimentation.