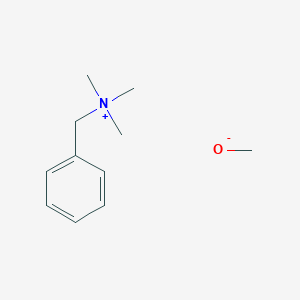
Benzyltrimethylammonium methoxide
Overview
Description
Benzyltrimethylammonium methoxide: is an organic compound with the molecular formula C11H19NO . It is a quaternary ammonium salt where the ammonium ion is substituted with a benzyl group and three methyl groups, and the counterion is methoxide. This compound is known for its use in organic synthesis and as a phase transfer catalyst.
Preparation Methods
Synthetic Routes and Reaction Conditions:
Reaction of Benzyl Chloride with Trimethylamine: One common method involves the reaction of benzyl chloride with trimethylamine in an anhydrous ethanol solution.
Electrolytic Method: Another method involves the electrolysis of benzyltrimethylammonium chloride in an aqueous solution to produce benzyltrimethylammonium hydroxide, which can then be converted to the methoxide form.
Industrial Production Methods: Industrial production typically follows the same synthetic routes but on a larger scale, with careful control of reaction conditions to ensure high yield and purity. The electrolytic method is particularly favored for its efficiency and high purity of the final product.
Chemical Reactions Analysis
Types of Reactions:
Substitution Reactions: Benzyltrimethylammonium methoxide can undergo nucleophilic substitution reactions where the methoxide ion acts as a nucleophile.
Elimination Reactions: It can also participate in elimination reactions, particularly in the formation of alkenes from alkyl halides.
Common Reagents and Conditions:
Nucleophilic Substitution: Typically involves reagents like alkyl halides under mild conditions.
Elimination Reactions: Often requires heat and a strong base.
Major Products:
Substitution Reactions: The major products are typically ethers or alcohols, depending on the substrate.
Elimination Reactions: The major products are alkenes.
Scientific Research Applications
Chemistry:
Catalysis: Used as a phase transfer catalyst in various organic reactions.
Synthesis: Employed in the synthesis of complex organic molecules due to its strong nucleophilic properties.
Biology and Medicine:
Biochemical Research: Utilized in the study of biochemical pathways and enzyme mechanisms.
Industry:
Polymerization: Acts as a catalyst in polymerization reactions.
Pharmaceuticals: Used in the synthesis of pharmaceutical intermediates.
Mechanism of Action
Mechanism: Benzyltrimethylammonium methoxide acts primarily through its methoxide ion, which is a strong nucleophile. It can attack electrophilic centers in organic molecules, facilitating substitution and elimination reactions.
Molecular Targets and Pathways:
Nucleophilic Attack: Targets electrophilic carbon atoms in substrates.
Catalysis: Facilitates the transfer of reactants between phases in phase transfer catalysis.
Comparison with Similar Compounds
Benzyltrimethylammonium hydroxide: Similar structure but with a hydroxide ion instead of methoxide.
Tetrabutylammonium methoxide: Another quaternary ammonium methoxide with butyl groups instead of benzyl.
Uniqueness:
Benzyl Group: The presence of the benzyl group in benzyltrimethylammonium methoxide provides unique reactivity and solubility properties compared to other quaternary ammonium methoxides.
Phase Transfer Catalysis: Its effectiveness as a phase transfer catalyst is enhanced by the benzyl group, making it more versatile in organic synthesis.
Properties
IUPAC Name |
benzyl(trimethyl)azanium;methanolate | |
---|---|---|
Source | PubChem | |
URL | https://pubchem.ncbi.nlm.nih.gov | |
Description | Data deposited in or computed by PubChem | |
InChI |
InChI=1S/C10H16N.CH3O/c1-11(2,3)9-10-7-5-4-6-8-10;1-2/h4-8H,9H2,1-3H3;1H3/q+1;-1 | |
Source | PubChem | |
URL | https://pubchem.ncbi.nlm.nih.gov | |
Description | Data deposited in or computed by PubChem | |
InChI Key |
SMTUJUHULKBTBS-UHFFFAOYSA-N | |
Source | PubChem | |
URL | https://pubchem.ncbi.nlm.nih.gov | |
Description | Data deposited in or computed by PubChem | |
Canonical SMILES |
C[N+](C)(C)CC1=CC=CC=C1.C[O-] | |
Source | PubChem | |
URL | https://pubchem.ncbi.nlm.nih.gov | |
Description | Data deposited in or computed by PubChem | |
Molecular Formula |
C11H19NO | |
Source | PubChem | |
URL | https://pubchem.ncbi.nlm.nih.gov | |
Description | Data deposited in or computed by PubChem | |
Related CAS |
14800-24-9 (Parent) | |
Record name | Benzyltrimethylammonium methoxide | |
Source | ChemIDplus | |
URL | https://pubchem.ncbi.nlm.nih.gov/substance/?source=chemidplus&sourceid=0000122087 | |
Description | ChemIDplus is a free, web search system that provides access to the structure and nomenclature authority files used for the identification of chemical substances cited in National Library of Medicine (NLM) databases, including the TOXNET system. | |
DSSTOX Substance ID |
DTXSID5059537 | |
Record name | Benzenemethanaminium, N,N,N-trimethyl-, methoxide | |
Source | EPA DSSTox | |
URL | https://comptox.epa.gov/dashboard/DTXSID5059537 | |
Description | DSSTox provides a high quality public chemistry resource for supporting improved predictive toxicology. | |
Molecular Weight |
181.27 g/mol | |
Source | PubChem | |
URL | https://pubchem.ncbi.nlm.nih.gov | |
Description | Data deposited in or computed by PubChem | |
Physical Description |
40% Methanol solution: Clear faintly yellow liquid; [Sigma-Aldrich MSDS] | |
Record name | Benzyltrimethylammonium methoxide | |
Source | Haz-Map, Information on Hazardous Chemicals and Occupational Diseases | |
URL | https://haz-map.com/Agents/20312 | |
Description | Haz-Map® is an occupational health database designed for health and safety professionals and for consumers seeking information about the adverse effects of workplace exposures to chemical and biological agents. | |
Explanation | Copyright (c) 2022 Haz-Map(R). All rights reserved. Unless otherwise indicated, all materials from Haz-Map are copyrighted by Haz-Map(R). No part of these materials, either text or image may be used for any purpose other than for personal use. Therefore, reproduction, modification, storage in a retrieval system or retransmission, in any form or by any means, electronic, mechanical or otherwise, for reasons other than personal use, is strictly prohibited without prior written permission. | |
CAS No. |
122-08-7 | |
Record name | Benzyltrimethylammonium methoxide | |
Source | CAS Common Chemistry | |
URL | https://commonchemistry.cas.org/detail?cas_rn=122-08-7 | |
Description | CAS Common Chemistry is an open community resource for accessing chemical information. Nearly 500,000 chemical substances from CAS REGISTRY cover areas of community interest, including common and frequently regulated chemicals, and those relevant to high school and undergraduate chemistry classes. This chemical information, curated by our expert scientists, is provided in alignment with our mission as a division of the American Chemical Society. | |
Explanation | The data from CAS Common Chemistry is provided under a CC-BY-NC 4.0 license, unless otherwise stated. | |
Record name | Benzyltrimethylammonium methoxide | |
Source | ChemIDplus | |
URL | https://pubchem.ncbi.nlm.nih.gov/substance/?source=chemidplus&sourceid=0000122087 | |
Description | ChemIDplus is a free, web search system that provides access to the structure and nomenclature authority files used for the identification of chemical substances cited in National Library of Medicine (NLM) databases, including the TOXNET system. | |
Record name | Benzyltrimethylammonium methoxide | |
Source | DTP/NCI | |
URL | https://dtp.cancer.gov/dtpstandard/servlet/dwindex?searchtype=NSC&outputformat=html&searchlist=221306 | |
Description | The NCI Development Therapeutics Program (DTP) provides services and resources to the academic and private-sector research communities worldwide to facilitate the discovery and development of new cancer therapeutic agents. | |
Explanation | Unless otherwise indicated, all text within NCI products is free of copyright and may be reused without our permission. Credit the National Cancer Institute as the source. | |
Record name | Benzenemethanaminium, N,N,N-trimethyl-, salt with methanol (1:1) | |
Source | EPA Chemicals under the TSCA | |
URL | https://www.epa.gov/chemicals-under-tsca | |
Description | EPA Chemicals under the Toxic Substances Control Act (TSCA) collection contains information on chemicals and their regulations under TSCA, including non-confidential content from the TSCA Chemical Substance Inventory and Chemical Data Reporting. | |
Record name | Benzenemethanaminium, N,N,N-trimethyl-, methoxide | |
Source | EPA DSSTox | |
URL | https://comptox.epa.gov/dashboard/DTXSID5059537 | |
Description | DSSTox provides a high quality public chemistry resource for supporting improved predictive toxicology. | |
Record name | Benzyltrimethylammonium methanolate | |
Source | European Chemicals Agency (ECHA) | |
URL | https://echa.europa.eu/substance-information/-/substanceinfo/100.004.111 | |
Description | The European Chemicals Agency (ECHA) is an agency of the European Union which is the driving force among regulatory authorities in implementing the EU's groundbreaking chemicals legislation for the benefit of human health and the environment as well as for innovation and competitiveness. | |
Explanation | Use of the information, documents and data from the ECHA website is subject to the terms and conditions of this Legal Notice, and subject to other binding limitations provided for under applicable law, the information, documents and data made available on the ECHA website may be reproduced, distributed and/or used, totally or in part, for non-commercial purposes provided that ECHA is acknowledged as the source: "Source: European Chemicals Agency, http://echa.europa.eu/". Such acknowledgement must be included in each copy of the material. ECHA permits and encourages organisations and individuals to create links to the ECHA website under the following cumulative conditions: Links can only be made to webpages that provide a link to the Legal Notice page. | |
Record name | Benzyltrimethylammonium methoxide | |
Source | FDA Global Substance Registration System (GSRS) | |
URL | https://gsrs.ncats.nih.gov/ginas/app/beta/substances/QT9DB9C7Y6 | |
Description | The FDA Global Substance Registration System (GSRS) enables the efficient and accurate exchange of information on what substances are in regulated products. Instead of relying on names, which vary across regulatory domains, countries, and regions, the GSRS knowledge base makes it possible for substances to be defined by standardized, scientific descriptions. | |
Explanation | Unless otherwise noted, the contents of the FDA website (www.fda.gov), both text and graphics, are not copyrighted. They are in the public domain and may be republished, reprinted and otherwise used freely by anyone without the need to obtain permission from FDA. Credit to the U.S. Food and Drug Administration as the source is appreciated but not required. | |
Retrosynthesis Analysis
AI-Powered Synthesis Planning: Our tool employs the Template_relevance Pistachio, Template_relevance Bkms_metabolic, Template_relevance Pistachio_ringbreaker, Template_relevance Reaxys, Template_relevance Reaxys_biocatalysis model, leveraging a vast database of chemical reactions to predict feasible synthetic routes.
One-Step Synthesis Focus: Specifically designed for one-step synthesis, it provides concise and direct routes for your target compounds, streamlining the synthesis process.
Accurate Predictions: Utilizing the extensive PISTACHIO, BKMS_METABOLIC, PISTACHIO_RINGBREAKER, REAXYS, REAXYS_BIOCATALYSIS database, our tool offers high-accuracy predictions, reflecting the latest in chemical research and data.
Strategy Settings
Precursor scoring | Relevance Heuristic |
---|---|
Min. plausibility | 0.01 |
Model | Template_relevance |
Template Set | Pistachio/Bkms_metabolic/Pistachio_ringbreaker/Reaxys/Reaxys_biocatalysis |
Top-N result to add to graph | 6 |
Feasible Synthetic Routes
Disclaimer and Information on In-Vitro Research Products
Please be aware that all articles and product information presented on BenchChem are intended solely for informational purposes. The products available for purchase on BenchChem are specifically designed for in-vitro studies, which are conducted outside of living organisms. In-vitro studies, derived from the Latin term "in glass," involve experiments performed in controlled laboratory settings using cells or tissues. It is important to note that these products are not categorized as medicines or drugs, and they have not received approval from the FDA for the prevention, treatment, or cure of any medical condition, ailment, or disease. We must emphasize that any form of bodily introduction of these products into humans or animals is strictly prohibited by law. It is essential to adhere to these guidelines to ensure compliance with legal and ethical standards in research and experimentation.