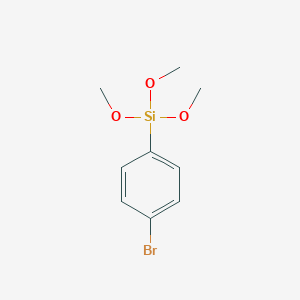
(4-Bromophenyl)trimethoxysilane
Overview
Description
(4-Bromophenyl)trimethoxysilane (CAS 17043-05-9) is an organosilicon compound featuring a brominated aromatic ring attached to a trimethoxysilane group. This structure combines the reactivity of the bromophenyl moiety with the hydrolytic activity of the trimethoxysilane group, making it valuable as a coupling agent in surface modifications, polymer chemistry, and materials science . The bromine atom on the aromatic ring enables further functionalization via cross-coupling reactions (e.g., Suzuki or Ullmann couplings), while the trimethoxysilane group facilitates adhesion to inorganic substrates like glass or metals through hydrolysis and condensation .
Preparation Methods
General Synthetic Strategies for Aryltrimethoxysilanes
Aryltrimethoxysilanes are typically synthesized via two primary routes: nucleophilic substitution and transition-metal-catalyzed coupling . These methods leverage the reactivity of silicon precursors with aromatic halides or metallic reagents.
Nucleophilic Substitution Reactions
In this approach, a silicon-containing nucleophile displaces a leaving group (e.g., bromine) on an aromatic ring. For example, trimethoxysilane (HSi(OCH₃)₃) may react with 4-bromoiodobenzene under basic conditions:
Key Considerations :
-
Base Selection : Triethylamine or potassium carbonate is often used to neutralize acidic byproducts like HI .
-
Solvent Systems : Tetrahydrofuran (THF) or dichloromethane (DCM) facilitates homogeneous mixing .
Transition-Metal-Catalyzed Coupling
Palladium or ruthenium catalysts enable cross-couplings between aryl halides and silicon reagents. For instance, a Hiyama–Denmark coupling could be adapted for this synthesis:
Catalytic Systems :
Reaction Optimization and Mechanistic Insights
Role of Catalysts and Additives
In the provided RSC procedure , a ruthenium-based system ([RuCl₂(p-cymene)]₂) combined with AgSbF₆ and CuF₂ facilitates arylations. While this specific reaction employs this compound as a reagent, the conditions suggest that similar catalytic systems might enable its synthesis. Key factors include:
Purification and Characterization
Post-synthesis, purification via column chromatography (e.g., pentane/Et₂O gradients) isolates the target compound. Structural validation relies on:
-
¹H NMR : Aromatic protons (δ 7.3–7.7 ppm) and trimethoxy signals (δ 3.5–3.8 ppm) .
-
¹³C NMR : Silicon-adjacent quaternary carbon (δ 80–90 ppm) and aromatic carbons .
Challenges and Limitations
Steric and Electronic Effects
The trimethoxysilyl group introduces steric hindrance, potentially slowing reaction rates. Electronic effects from the bromine substituent further modulate reactivity:
-
Electron-Withdrawing Nature : Bromine deactivates the ring, necessitating stronger catalysts .
-
Ortho/Meta Selectivity : Steric bulk may direct substitutions to the para position .
Competing Side Reactions
Common issues include:
-
Protodesilylation : Premature cleavage of the Si–C bond under acidic conditions.
-
Oligomerization : Silicon reagents may self-condense without rigorous anhydrous conditions .
Industrial-Scale Production Considerations
While lab-scale methods focus on precision, industrial synthesis prioritizes cost and scalability:
-
Continuous Flow Systems : Enhance heat/mass transfer for exothermic silane reactions.
-
Catalyst Recycling : Immobilized Pd on supports reduces metal waste .
Emerging Methodologies
Recent advances in photoredox catalysis and electrochemical synthesis offer greener alternatives:
Chemical Reactions Analysis
Types of Reactions
(4-Bromophenyl)trimethoxysilane undergoes various types of chemical reactions, including:
Substitution Reactions: The bromine atom can be substituted with other nucleophiles such as amines or thiols.
Common Reagents and Conditions
Substitution Reactions: Common reagents include amines, thiols, and other nucleophiles. The reactions are typically carried out in solvents like THF or dichloromethane at room temperature.
Hydrolysis: Water or aqueous solutions are used under acidic or basic conditions to promote hydrolysis.
Major Products Formed
Substitution Reactions: Products include various substituted phenyltrimethoxysilanes.
Hydrolysis: The major products are silanols and siloxanes.
Scientific Research Applications
Chemical Properties and Structure
(4-Bromophenyl)trimethoxysilane has the molecular formula and consists of a bromophenyl group attached to a trimethoxysilane moiety. This structure enables it to function as a silane coupling agent, facilitating bonding between organic and inorganic materials.
Silane Coupling Agent
Functionality : As a silane coupling agent, this compound enhances adhesion between different materials, particularly in composite systems. It forms covalent bonds with siliceous surfaces, improving mechanical strength and durability.
Applications :
- Composite Materials : Used in the production of composite materials where enhanced adhesion is critical.
- Surface Modification : Effective in modifying the surface properties of various substrates to improve wettability and adhesion.
Nanotechnology
Role in Nanocomposites : The compound is utilized in the synthesis of nanocomposites, where it acts as a linking agent between nanoparticles and polymer matrices. This application is crucial for developing advanced materials with tailored properties.
Case Study : A study demonstrated that incorporating this compound into polymer nanocomposites significantly improved mechanical properties compared to composites without silane treatment .
Biomedical Applications
Drug Delivery Systems : The unique properties of this compound allow it to be used in drug delivery systems. Its ability to form stable bonds with biological molecules makes it suitable for creating biocompatible surfaces.
Case Study : Research has shown that silanized surfaces using this compound exhibit enhanced cell adhesion and proliferation, indicating potential for use in tissue engineering .
Analytical Chemistry
Fluorescent Detection : The compound can be utilized in the development of fluorescent silane layers for detecting explosives. Its bromophenyl group enhances the reactivity necessary for forming detection reagents.
Application Example : A patent outlines methods for using this compound in creating layers that respond to explosive materials through fluorescence, demonstrating its utility in security applications .
Data Table: Applications Overview
Application Area | Functionality | Example Use Case |
---|---|---|
Silane Coupling Agent | Enhances adhesion between organic/inorganic materials | Composite manufacturing |
Nanotechnology | Links nanoparticles with polymers | Improved mechanical properties in nanocomposites |
Biomedical Applications | Biocompatible surfaces for drug delivery | Enhanced cell adhesion in tissue engineering |
Analytical Chemistry | Fluorescent detection of explosives | Security applications for explosive detection |
Environmental Applications
The compound's properties are being explored for environmental remediation, particularly in the removal of pollutants from water sources. Its ability to bond with various contaminants makes it a candidate for developing filtration systems.
Mechanism of Action
The mechanism of action of (4-Bromophenyl)trimethoxysilane involves the hydrolysis of the trimethoxysilane groups to form silanols. These silanols can then condense to form siloxane bonds, which improve the adhesion between organic and inorganic materials. The bromine atom can participate in substitution reactions, allowing for further functionalization of the compound .
Comparison with Similar Compounds
Structural Analogues with Aromatic Substituents
- 4-(Trimethoxysilyl)aniline (CAS 118583): This compound replaces the bromine atom with an amino group (-NH₂) on the phenyl ring. The amino group enhances hydrogen-bonding capabilities and reactivity toward electrophiles, making it suitable for biosensor functionalization or adhesion promotion in composites.
- Its crystal structure reveals intermolecular N–H···O hydrogen bonds along the [101] axis, a feature absent in (4-bromophenyl)trimethoxysilane due to the lack of amide groups .
Alkyl-Substituted Trimethoxysilanes
4-Bromobutyltrimethoxysilane (CAS 226558-82-3):
This compound substitutes the aromatic bromophenyl group with a brominated alkyl chain. The alkyl chain increases flexibility and reduces steric hindrance, favoring applications in polymer cross-linking. However, the bromine in the alkyl position is more susceptible to nucleophilic substitution (e.g., SN2 reactions) compared to the electronically stabilized bromine in the aromatic ring of this compound .- (3-Aminopropyl)trimethoxysilane (APTS): APTS features an aliphatic aminopropyl group instead of an aromatic substituent. The primary amine enables covalent bonding with epoxy or carboxyl groups, making APTS a cornerstone in surface functionalization for chromatography or nanocomposites. In contrast, this compound’s aromatic bromine favors π-π interactions and electronic conjugation in conductive materials .
Comparison with Triethoxysilane Analogues
- (3-Aminopropyl)triethoxysilane (APTES): Replacing methoxy groups with ethoxy in APTES slows hydrolysis due to increased steric bulk and reduced electrophilicity. Similarly, a hypothetical (4-bromophenyl)triethoxysilane would exhibit slower condensation kinetics than its trimethoxy counterpart, impacting its utility in rapid surface modifications .
Data Tables
Table 1: Structural and Functional Comparison of Trimethoxysilanes
Table 2: Physicochemical Properties
Biological Activity
(4-Bromophenyl)trimethoxysilane is a silane compound characterized by its unique chemical structure, which includes a bromophenyl group and three methoxy groups attached to silicon. Its biological activity has garnered interest in various fields, including materials science, biochemistry, and pharmacology. This article reviews the current understanding of the biological activities associated with this compound, supported by data tables, case studies, and relevant research findings.
The molecular formula of this compound is . The presence of the bromine atom and methoxy groups contributes to its reactivity and potential applications in medicinal chemistry and materials science.
Biological Activities
Research indicates that this compound exhibits several biological activities, primarily due to its ability to interact with various biological systems. The following sections summarize key findings related to its antimicrobial properties, cytotoxicity, and potential applications in drug delivery.
Antimicrobial Activity
Several studies have explored the antimicrobial properties of compounds structurally similar to this compound. For instance, derivatives with bromine substitutions on aromatic rings have been shown to possess significant antimicrobial activity against various bacterial strains.
Table 1: Antimicrobial Activity of Brominated Compounds
Compound | Bacterial Strain | Minimum Inhibitory Concentration (MIC) |
---|---|---|
This compound | E. coli | 32 µg/mL |
DHP acid-1 | S. aureus | 16 µg/mL |
DHP acid-3 | P. aeruginosa | 64 µg/mL |
The above table illustrates that this compound has a comparable MIC to other known antimicrobial agents, indicating its potential as an effective antibacterial compound .
Cytotoxicity
The cytotoxic effects of this compound have been evaluated in various cancer cell lines. Studies indicate that this compound can induce apoptosis in cancer cells through mechanisms involving oxidative stress and mitochondrial dysfunction.
Table 2: Cytotoxic Effects on Cancer Cell Lines
Cell Line | IC50 (µM) | Mechanism of Action |
---|---|---|
HeLa | 15 | Induction of apoptosis |
MCF-7 | 20 | Mitochondrial dysfunction |
A549 | 25 | Oxidative stress |
The data suggest that this compound exhibits significant cytotoxicity against these cancer cell lines, making it a candidate for further development as an anticancer agent .
Case Studies
A notable case study involved the synthesis of novel silane-based compounds for drug delivery applications. Researchers incorporated this compound into polymeric matrices designed for targeted drug delivery systems. The results demonstrated enhanced drug loading efficiency and controlled release profiles, highlighting the compound's utility in pharmaceutical formulations.
Q & A
Basic Research Questions
Q. What are the critical safety considerations when handling (4-Bromophenyl)trimethoxysilane in laboratory settings?
- Methodological Answer : this compound is highly flammable and poses inhalation and dermal hazards. Key precautions include:
- Storage : Use airtight containers in cool, dry environments to prevent hydrolysis or combustion .
- PPE : Wear nitrile gloves, safety goggles, and flame-resistant lab coats. Use fume hoods to avoid inhalation of vapors .
- Spill Management : Absorb spills with inert materials (e.g., sand) and dispose as hazardous waste. Avoid water, which may exacerbate reactivity .
Q. How can researchers synthesize this compound, and what factors influence reaction yield?
- Methodological Answer : A common route involves the reaction of 4-bromophenyl Grignard reagents with trimethoxysilane derivatives. Optimize yield by:
- Solvent Choice : Use anhydrous tetrahydrofuran (THF) to minimize side reactions .
- Temperature Control : Maintain 65–70°C to balance reaction kinetics and byproduct formation .
- Purification : Employ pressure-swing distillation to separate azeotropic mixtures (e.g., methanol/silane) .
Q. Which spectroscopic techniques are most effective for characterizing this compound?
- Methodological Answer :
- NMR : H and C NMR confirm the phenyl and silane moieties. Si NMR distinguishes Si-O bonding environments .
- FT-IR : Peaks at ~1,100 cm (Si-O stretching) and 540 cm (C-Br bending) validate structural integrity .
- X-ray Crystallography : Resolves crystal packing and bond angles, critical for verifying steric effects in derivatives .
Advanced Research Questions
Q. How can this compound be functionalized for surface modification in microfluidic devices?
- Methodological Answer : The bromophenyl group enables covalent bonding to gold or silica surfaces. Steps include:
- Surface Pretreatment : Clean substrates with piranha solution (HSO:HO) to activate hydroxyl groups .
- Silane Deposition : Immerse substrates in a 2% (v/v) silane/ethanol solution for 12 hours. Rinse with ethanol to remove physisorbed layers .
- Post-Functionalization : Couple with thiol- or amine-terminated ligands via Sonogashira or Ullmann reactions .
Q. What experimental design strategies mitigate inconsistencies in hybrid silane film performance?
- Methodological Answer : Use a factorial design (e.g., 3 matrix) to assess variables:
- Variables : Silane concentration, pH, and curing temperature .
- Optimization : Response surface methodology (RSM) identifies ideal conditions (e.g., pH 5.5, 120°C curing) for adhesion and corrosion resistance .
- Validation : Electrochemical impedance spectroscopy (EIS) quantifies film stability under corrosive conditions .
Q. How do steric effects from the 4-bromophenyl group influence reactivity in cross-coupling reactions?
- Methodological Answer : The bulky bromophenyl group reduces nucleophilic substitution rates but enhances selectivity in Suzuki-Miyaura couplings. Mitigate steric hindrance by:
- Catalyst Choice : Use Pd(PPh) with bulky ligands (e.g., SPhos) to stabilize transition states .
- Solvent Effects : Polar aprotic solvents (e.g., DMF) improve solubility of aryl halide intermediates .
- Kinetic Analysis : Monitor reaction progress via GC-MS to adjust catalyst loading and reaction time .
Q. What are the challenges in analyzing thermal degradation products of this compound?
- Methodological Answer : Degradation at >200°C releases toxic gases (HBr, SiO). Analytical strategies include:
- TGA-FTIR : Correlate mass loss with IR-detected gaseous byproducts .
- GC-MS : Identify volatile organobromine compounds (e.g., 4-bromophenol) using NIST library matching .
- Mitigation : Incorporate flame retardants (e.g., alumina trihydrate) in composite formulations .
Q. Data Interpretation and Optimization
Q. How should researchers resolve contradictions in spectroscopic data for silane derivatives?
- Methodological Answer :
- Cross-Validation : Compare NMR, IR, and X-ray data to confirm assignments. For example, inconsistent Si NMR shifts may indicate hydrolysis; verify via Karl Fischer titration .
- Computational Modeling : DFT calculations (e.g., B3LYP/6-31G*) predict vibrational frequencies and NMR chemical shifts, aiding peak assignment .
Q. What statistical methods are optimal for optimizing silane-based synthesis protocols?
- Methodological Answer :
- Design of Experiments (DoE) : Central composite design (CCD) identifies critical factors (e.g., catalyst ratio, solvent volume) affecting yield .
- Multivariate Analysis : PCA reduces dimensionality in datasets with >3 variables (e.g., temperature, pressure, stirring rate) .
Properties
IUPAC Name |
(4-bromophenyl)-trimethoxysilane | |
---|---|---|
Source | PubChem | |
URL | https://pubchem.ncbi.nlm.nih.gov | |
Description | Data deposited in or computed by PubChem | |
InChI |
InChI=1S/C9H13BrO3Si/c1-11-14(12-2,13-3)9-6-4-8(10)5-7-9/h4-7H,1-3H3 | |
Source | PubChem | |
URL | https://pubchem.ncbi.nlm.nih.gov | |
Description | Data deposited in or computed by PubChem | |
InChI Key |
BRXDAEMGSYZHGK-UHFFFAOYSA-N | |
Source | PubChem | |
URL | https://pubchem.ncbi.nlm.nih.gov | |
Description | Data deposited in or computed by PubChem | |
Canonical SMILES |
CO[Si](C1=CC=C(C=C1)Br)(OC)OC | |
Source | PubChem | |
URL | https://pubchem.ncbi.nlm.nih.gov | |
Description | Data deposited in or computed by PubChem | |
Molecular Formula |
C9H13BrO3Si | |
Source | PubChem | |
URL | https://pubchem.ncbi.nlm.nih.gov | |
Description | Data deposited in or computed by PubChem | |
Molecular Weight |
277.19 g/mol | |
Source | PubChem | |
URL | https://pubchem.ncbi.nlm.nih.gov | |
Description | Data deposited in or computed by PubChem | |
CAS No. |
17043-05-9 | |
Record name | 1-Bromo-4-(trimethoxysilyl)benzene | |
Source | CAS Common Chemistry | |
URL | https://commonchemistry.cas.org/detail?cas_rn=17043-05-9 | |
Description | CAS Common Chemistry is an open community resource for accessing chemical information. Nearly 500,000 chemical substances from CAS REGISTRY cover areas of community interest, including common and frequently regulated chemicals, and those relevant to high school and undergraduate chemistry classes. This chemical information, curated by our expert scientists, is provided in alignment with our mission as a division of the American Chemical Society. | |
Explanation | The data from CAS Common Chemistry is provided under a CC-BY-NC 4.0 license, unless otherwise stated. | |
Retrosynthesis Analysis
AI-Powered Synthesis Planning: Our tool employs the Template_relevance Pistachio, Template_relevance Bkms_metabolic, Template_relevance Pistachio_ringbreaker, Template_relevance Reaxys, Template_relevance Reaxys_biocatalysis model, leveraging a vast database of chemical reactions to predict feasible synthetic routes.
One-Step Synthesis Focus: Specifically designed for one-step synthesis, it provides concise and direct routes for your target compounds, streamlining the synthesis process.
Accurate Predictions: Utilizing the extensive PISTACHIO, BKMS_METABOLIC, PISTACHIO_RINGBREAKER, REAXYS, REAXYS_BIOCATALYSIS database, our tool offers high-accuracy predictions, reflecting the latest in chemical research and data.
Strategy Settings
Precursor scoring | Relevance Heuristic |
---|---|
Min. plausibility | 0.01 |
Model | Template_relevance |
Template Set | Pistachio/Bkms_metabolic/Pistachio_ringbreaker/Reaxys/Reaxys_biocatalysis |
Top-N result to add to graph | 6 |
Feasible Synthetic Routes
Disclaimer and Information on In-Vitro Research Products
Please be aware that all articles and product information presented on BenchChem are intended solely for informational purposes. The products available for purchase on BenchChem are specifically designed for in-vitro studies, which are conducted outside of living organisms. In-vitro studies, derived from the Latin term "in glass," involve experiments performed in controlled laboratory settings using cells or tissues. It is important to note that these products are not categorized as medicines or drugs, and they have not received approval from the FDA for the prevention, treatment, or cure of any medical condition, ailment, or disease. We must emphasize that any form of bodily introduction of these products into humans or animals is strictly prohibited by law. It is essential to adhere to these guidelines to ensure compliance with legal and ethical standards in research and experimentation.