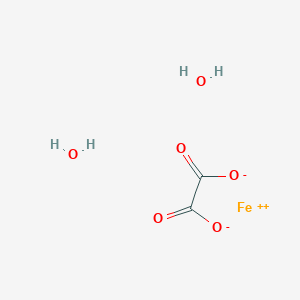
Ferrous oxalate dihydrate
Overview
Description
Ferrous oxalate dihydrate, also known as iron(II) oxalate dihydrate, is a coordination polymer with the chemical formula FeC₂O₄·2H₂O. It consists of chains of oxalate-bridged ferrous centers, each capped by water molecules. This compound is known for its yellowish appearance and is poorly soluble in water. It is commonly used in various scientific and industrial applications due to its unique properties .
Mechanism of Action
Target of Action
Ferrous oxalate dihydrate (FeC2O4·2H2O) is a coordination polymer that consists of chains of oxalate-bridged ferrous centers, each capped by water molecules . It primarily targets the photocatalytic and Fenton oxidation processes . It has been successfully prepared for use as a bifunctional catalyst in both photocatalysis and Fenton oxidation .
Mode of Action
This compound interacts with its targets through a unique two-pathway photocatalytic mechanism which combines traditional photocatalytic and photo-initiated Fenton oxidations . The calculation results indicated that N,N-Diethylacetamide (DEAc) molecules can affect the growth of this compound crystals by hydrogen bonding and van der Waals forces . DEAc encases this compound crystals to inhibit crystal growth, and preferentially binds to both sides of C2O4^2- to control the axial growth of this compound crystals .
Biochemical Pathways
The biochemical pathways affected by this compound are primarily related to the degradation of organic pollutants. It has been demonstrated that this compound exhibits significant Fenton oxidation activity in degrading organic compounds in the presence of H2O2 in the dark . Moreover, it has remarkable n-type semiconductor characteristics, which contribute to its extraordinary photocatalytic activities .
Result of Action
The primary result of this compound’s action is the degradation of organic pollutants. For instance, it has been shown to reduce Cr(VI) into Cr(III) under visible light irradiation . When heated, it dehydrates and decomposes into iron oxides and a pyrophoric black iron, and it also evolves carbon dioxide and carbon monoxide gases .
Action Environment
The action, efficacy, and stability of this compound can be influenced by various environmental factors. For example, the presence of light can enhance its photocatalytic activity . Moreover, the addition of certain molecules like DEAc can affect the growth of this compound crystals, thereby influencing its overall activity .
Preparation Methods
Synthetic Routes and Reaction Conditions: Ferrous oxalate dihydrate can be synthesized through several methods. One common approach involves the reaction of ferrous sulfate with oxalic acid in an aqueous medium. The reaction typically occurs at elevated temperatures to ensure complete precipitation of the this compound. Another method involves the use of ferrotitaniferous mineral sands and hot pressurized aqueous oxalic acid. This method has been shown to yield high purity this compound with significant reaction rates and yields .
Industrial Production Methods: In industrial settings, this compound is often produced using large-scale precipitation methods. The process involves dissolving ferrous salts in water, followed by the addition of oxalic acid. The resulting precipitate is then filtered, washed, and dried to obtain the final product. This method is efficient and cost-effective, making it suitable for large-scale production .
Chemical Reactions Analysis
Types of Reactions: Ferrous oxalate dihydrate undergoes several types of chemical reactions, including:
Reduction: It can be reduced to metallic iron under specific conditions.
Substitution: this compound can react with other ligands to form different coordination compounds.
Common Reagents and Conditions:
Oxidation: Heating this compound to temperatures around 190°C results in its decomposition.
Reduction: Reducing agents such as hydrogen gas can be used to convert this compound to metallic iron.
Substitution: Ligands such as ammonia or phosphates can be used to substitute the oxalate ligands in this compound.
Major Products Formed:
Oxidation: Iron oxides (e.g., Fe₂O₃) and gases (CO₂ and CO).
Reduction: Metallic iron.
Substitution: Various coordination compounds depending on the substituting ligand.
Scientific Research Applications
Ferrous oxalate dihydrate has a wide range of applications in scientific research:
Biology: this compound is studied for its potential use in biological systems, particularly in the context of iron metabolism and transport.
Medicine: Research is ongoing to explore its potential therapeutic applications, including its use in drug delivery systems.
Comparison with Similar Compounds
Ferrous oxalate dihydrate can be compared with other similar compounds such as:
Ferric oxalate: Unlike this compound, ferric oxalate contains iron in the +3 oxidation state and has different chemical properties and reactivity.
Potassium ferrioxalate: This compound is a coordination complex of iron(III) with oxalate and potassium ions, and it is used in photochemical studies.
Sodium ferrioxalate: Similar to potassium ferrioxalate, it is used in photochemical applications but has different solubility and stability properties.
Uniqueness: this compound is unique due to its ability to act as a bifunctional catalyst in both photocatalysis and Fenton oxidation. Its structure allows for efficient electron transfer, making it highly effective in degrading organic pollutants under visible light irradiation .
Properties
IUPAC Name |
iron(2+);oxalate;dihydrate | |
---|---|---|
Source | PubChem | |
URL | https://pubchem.ncbi.nlm.nih.gov | |
Description | Data deposited in or computed by PubChem | |
InChI |
InChI=1S/C2H2O4.Fe.2H2O/c3-1(4)2(5)6;;;/h(H,3,4)(H,5,6);;2*1H2/q;+2;;/p-2 | |
Source | PubChem | |
URL | https://pubchem.ncbi.nlm.nih.gov | |
Description | Data deposited in or computed by PubChem | |
InChI Key |
NPLZZSLZTJVZSX-UHFFFAOYSA-L | |
Source | PubChem | |
URL | https://pubchem.ncbi.nlm.nih.gov | |
Description | Data deposited in or computed by PubChem | |
Canonical SMILES |
C(=O)(C(=O)[O-])[O-].O.O.[Fe+2] | |
Source | PubChem | |
URL | https://pubchem.ncbi.nlm.nih.gov | |
Description | Data deposited in or computed by PubChem | |
Molecular Formula |
C2H4FeO6 | |
Source | PubChem | |
URL | https://pubchem.ncbi.nlm.nih.gov | |
Description | Data deposited in or computed by PubChem | |
DSSTOX Substance ID |
DTXSID90975879 | |
Record name | Iron(2+) ethanedioate--water (1/1/2) | |
Source | EPA DSSTox | |
URL | https://comptox.epa.gov/dashboard/DTXSID90975879 | |
Description | DSSTox provides a high quality public chemistry resource for supporting improved predictive toxicology. | |
Molecular Weight |
179.89 g/mol | |
Source | PubChem | |
URL | https://pubchem.ncbi.nlm.nih.gov | |
Description | Data deposited in or computed by PubChem | |
CAS No. |
6047-25-2 | |
Record name | Ferrous oxalate dihydrate | |
Source | CAS Common Chemistry | |
URL | https://commonchemistry.cas.org/detail?cas_rn=6047-25-2 | |
Description | CAS Common Chemistry is an open community resource for accessing chemical information. Nearly 500,000 chemical substances from CAS REGISTRY cover areas of community interest, including common and frequently regulated chemicals, and those relevant to high school and undergraduate chemistry classes. This chemical information, curated by our expert scientists, is provided in alignment with our mission as a division of the American Chemical Society. | |
Explanation | The data from CAS Common Chemistry is provided under a CC-BY-NC 4.0 license, unless otherwise stated. | |
Record name | Ferrous oxalate dihydrate | |
Source | ChemIDplus | |
URL | https://pubchem.ncbi.nlm.nih.gov/substance/?source=chemidplus&sourceid=0006047252 | |
Description | ChemIDplus is a free, web search system that provides access to the structure and nomenclature authority files used for the identification of chemical substances cited in National Library of Medicine (NLM) databases, including the TOXNET system. | |
Record name | Iron(2+) ethanedioate--water (1/1/2) | |
Source | EPA DSSTox | |
URL | https://comptox.epa.gov/dashboard/DTXSID90975879 | |
Description | DSSTox provides a high quality public chemistry resource for supporting improved predictive toxicology. | |
Record name | Iron, diaqua[ethanedioato(2-)-κO1,κO2]-, (T-4) | |
Source | European Chemicals Agency (ECHA) | |
URL | https://echa.europa.eu/substance-information/-/substanceinfo/100.109.814 | |
Description | The European Chemicals Agency (ECHA) is an agency of the European Union which is the driving force among regulatory authorities in implementing the EU's groundbreaking chemicals legislation for the benefit of human health and the environment as well as for innovation and competitiveness. | |
Explanation | Use of the information, documents and data from the ECHA website is subject to the terms and conditions of this Legal Notice, and subject to other binding limitations provided for under applicable law, the information, documents and data made available on the ECHA website may be reproduced, distributed and/or used, totally or in part, for non-commercial purposes provided that ECHA is acknowledged as the source: "Source: European Chemicals Agency, http://echa.europa.eu/". Such acknowledgement must be included in each copy of the material. ECHA permits and encourages organisations and individuals to create links to the ECHA website under the following cumulative conditions: Links can only be made to webpages that provide a link to the Legal Notice page. | |
Record name | FERROUS OXALATE DIHYDRATE | |
Source | FDA Global Substance Registration System (GSRS) | |
URL | https://gsrs.ncats.nih.gov/ginas/app/beta/substances/Z6X3YBU50D | |
Description | The FDA Global Substance Registration System (GSRS) enables the efficient and accurate exchange of information on what substances are in regulated products. Instead of relying on names, which vary across regulatory domains, countries, and regions, the GSRS knowledge base makes it possible for substances to be defined by standardized, scientific descriptions. | |
Explanation | Unless otherwise noted, the contents of the FDA website (www.fda.gov), both text and graphics, are not copyrighted. They are in the public domain and may be republished, reprinted and otherwise used freely by anyone without the need to obtain permission from FDA. Credit to the U.S. Food and Drug Administration as the source is appreciated but not required. | |
Synthesis routes and methods
Procedure details
Retrosynthesis Analysis
AI-Powered Synthesis Planning: Our tool employs the Template_relevance Pistachio, Template_relevance Bkms_metabolic, Template_relevance Pistachio_ringbreaker, Template_relevance Reaxys, Template_relevance Reaxys_biocatalysis model, leveraging a vast database of chemical reactions to predict feasible synthetic routes.
One-Step Synthesis Focus: Specifically designed for one-step synthesis, it provides concise and direct routes for your target compounds, streamlining the synthesis process.
Accurate Predictions: Utilizing the extensive PISTACHIO, BKMS_METABOLIC, PISTACHIO_RINGBREAKER, REAXYS, REAXYS_BIOCATALYSIS database, our tool offers high-accuracy predictions, reflecting the latest in chemical research and data.
Strategy Settings
Precursor scoring | Relevance Heuristic |
---|---|
Min. plausibility | 0.01 |
Model | Template_relevance |
Template Set | Pistachio/Bkms_metabolic/Pistachio_ringbreaker/Reaxys/Reaxys_biocatalysis |
Top-N result to add to graph | 6 |
Feasible Synthetic Routes
Disclaimer and Information on In-Vitro Research Products
Please be aware that all articles and product information presented on BenchChem are intended solely for informational purposes. The products available for purchase on BenchChem are specifically designed for in-vitro studies, which are conducted outside of living organisms. In-vitro studies, derived from the Latin term "in glass," involve experiments performed in controlled laboratory settings using cells or tissues. It is important to note that these products are not categorized as medicines or drugs, and they have not received approval from the FDA for the prevention, treatment, or cure of any medical condition, ailment, or disease. We must emphasize that any form of bodily introduction of these products into humans or animals is strictly prohibited by law. It is essential to adhere to these guidelines to ensure compliance with legal and ethical standards in research and experimentation.