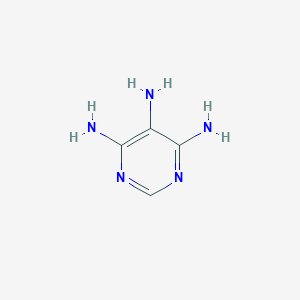
4,5,6-Triaminopyrimidine
Overview
Description
4,5,6-Triaminopyrimidine (TAP) is an organic compound that has been studied extensively in scientific research due to its potential applications in the field of biochemistry and physiology. TAP is a heterocyclic aromatic compound, which means that it contains a ring of atoms with alternating single and double bonds. It is also an aminopyrimidine, which means that it contains an amine group attached to a pyrimidine ring. TAP is a very versatile compound, and its properties have been used in a variety of scientific research applications.
Safety and Hazards
When handling 4,5,6-Triaminopyrimidine, it is recommended to avoid dust formation, breathing mist, gas or vapours, and contact with skin and eyes . Personal protective equipment should be used, including chemical impermeable gloves . Adequate ventilation should be ensured, and all sources of ignition should be removed .
Future Directions
properties
IUPAC Name |
pyrimidine-4,5,6-triamine | |
---|---|---|
Source | PubChem | |
URL | https://pubchem.ncbi.nlm.nih.gov | |
Description | Data deposited in or computed by PubChem | |
InChI |
InChI=1S/C4H7N5/c5-2-3(6)8-1-9-4(2)7/h1H,5H2,(H4,6,7,8,9) | |
Source | PubChem | |
URL | https://pubchem.ncbi.nlm.nih.gov | |
Description | Data deposited in or computed by PubChem | |
InChI Key |
MPNBXFXEMHPGTK-UHFFFAOYSA-N | |
Source | PubChem | |
URL | https://pubchem.ncbi.nlm.nih.gov | |
Description | Data deposited in or computed by PubChem | |
Canonical SMILES |
C1=NC(=C(C(=N1)N)N)N | |
Source | PubChem | |
URL | https://pubchem.ncbi.nlm.nih.gov | |
Description | Data deposited in or computed by PubChem | |
Molecular Formula |
C4H7N5 | |
Source | PubChem | |
URL | https://pubchem.ncbi.nlm.nih.gov | |
Description | Data deposited in or computed by PubChem | |
Related CAS |
49721-45-1 (sulfate 1:1), 6640-23-9 (sulfate 1:1 monohydrate), 68738-86-3 (sulfate) | |
Record name | 4,5,6-Triaminopyrimidine | |
Source | ChemIDplus | |
URL | https://pubchem.ncbi.nlm.nih.gov/substance/?source=chemidplus&sourceid=0000118707 | |
Description | ChemIDplus is a free, web search system that provides access to the structure and nomenclature authority files used for the identification of chemical substances cited in National Library of Medicine (NLM) databases, including the TOXNET system. | |
DSSTOX Substance ID |
DTXSID10151996 | |
Record name | 4,5,6-Triaminopyrimidine | |
Source | EPA DSSTox | |
URL | https://comptox.epa.gov/dashboard/DTXSID10151996 | |
Description | DSSTox provides a high quality public chemistry resource for supporting improved predictive toxicology. | |
Molecular Weight |
125.13 g/mol | |
Source | PubChem | |
URL | https://pubchem.ncbi.nlm.nih.gov | |
Description | Data deposited in or computed by PubChem | |
CAS RN |
118-70-7 | |
Record name | 4,5,6-Pyrimidinetriamine | |
Source | CAS Common Chemistry | |
URL | https://commonchemistry.cas.org/detail?cas_rn=118-70-7 | |
Description | CAS Common Chemistry is an open community resource for accessing chemical information. Nearly 500,000 chemical substances from CAS REGISTRY cover areas of community interest, including common and frequently regulated chemicals, and those relevant to high school and undergraduate chemistry classes. This chemical information, curated by our expert scientists, is provided in alignment with our mission as a division of the American Chemical Society. | |
Explanation | The data from CAS Common Chemistry is provided under a CC-BY-NC 4.0 license, unless otherwise stated. | |
Record name | 4,5,6-Triaminopyrimidine | |
Source | ChemIDplus | |
URL | https://pubchem.ncbi.nlm.nih.gov/substance/?source=chemidplus&sourceid=0000118707 | |
Description | ChemIDplus is a free, web search system that provides access to the structure and nomenclature authority files used for the identification of chemical substances cited in National Library of Medicine (NLM) databases, including the TOXNET system. | |
Record name | 118-70-7 | |
Source | DTP/NCI | |
URL | https://dtp.cancer.gov/dtpstandard/servlet/dwindex?searchtype=NSC&outputformat=html&searchlist=145059 | |
Description | The NCI Development Therapeutics Program (DTP) provides services and resources to the academic and private-sector research communities worldwide to facilitate the discovery and development of new cancer therapeutic agents. | |
Explanation | Unless otherwise indicated, all text within NCI products is free of copyright and may be reused without our permission. Credit the National Cancer Institute as the source. | |
Record name | 4,5,6-Triaminopyrimidine | |
Source | EPA DSSTox | |
URL | https://comptox.epa.gov/dashboard/DTXSID10151996 | |
Description | DSSTox provides a high quality public chemistry resource for supporting improved predictive toxicology. | |
Record name | Pyrimidine-4,5,6-triyltriamine | |
Source | European Chemicals Agency (ECHA) | |
URL | https://echa.europa.eu/substance-information/-/substanceinfo/100.003.883 | |
Description | The European Chemicals Agency (ECHA) is an agency of the European Union which is the driving force among regulatory authorities in implementing the EU's groundbreaking chemicals legislation for the benefit of human health and the environment as well as for innovation and competitiveness. | |
Explanation | Use of the information, documents and data from the ECHA website is subject to the terms and conditions of this Legal Notice, and subject to other binding limitations provided for under applicable law, the information, documents and data made available on the ECHA website may be reproduced, distributed and/or used, totally or in part, for non-commercial purposes provided that ECHA is acknowledged as the source: "Source: European Chemicals Agency, http://echa.europa.eu/". Such acknowledgement must be included in each copy of the material. ECHA permits and encourages organisations and individuals to create links to the ECHA website under the following cumulative conditions: Links can only be made to webpages that provide a link to the Legal Notice page. | |
Record name | 4,5,6-TRIAMINOPYRIMIDINE | |
Source | FDA Global Substance Registration System (GSRS) | |
URL | https://gsrs.ncats.nih.gov/ginas/app/beta/substances/PHL9UQ3Q4H | |
Description | The FDA Global Substance Registration System (GSRS) enables the efficient and accurate exchange of information on what substances are in regulated products. Instead of relying on names, which vary across regulatory domains, countries, and regions, the GSRS knowledge base makes it possible for substances to be defined by standardized, scientific descriptions. | |
Explanation | Unless otherwise noted, the contents of the FDA website (www.fda.gov), both text and graphics, are not copyrighted. They are in the public domain and may be republished, reprinted and otherwise used freely by anyone without the need to obtain permission from FDA. Credit to the U.S. Food and Drug Administration as the source is appreciated but not required. | |
Retrosynthesis Analysis
AI-Powered Synthesis Planning: Our tool employs the Template_relevance Pistachio, Template_relevance Bkms_metabolic, Template_relevance Pistachio_ringbreaker, Template_relevance Reaxys, Template_relevance Reaxys_biocatalysis model, leveraging a vast database of chemical reactions to predict feasible synthetic routes.
One-Step Synthesis Focus: Specifically designed for one-step synthesis, it provides concise and direct routes for your target compounds, streamlining the synthesis process.
Accurate Predictions: Utilizing the extensive PISTACHIO, BKMS_METABOLIC, PISTACHIO_RINGBREAKER, REAXYS, REAXYS_BIOCATALYSIS database, our tool offers high-accuracy predictions, reflecting the latest in chemical research and data.
Strategy Settings
Precursor scoring | Relevance Heuristic |
---|---|
Min. plausibility | 0.01 |
Model | Template_relevance |
Template Set | Pistachio/Bkms_metabolic/Pistachio_ringbreaker/Reaxys/Reaxys_biocatalysis |
Top-N result to add to graph | 6 |
Feasible Synthetic Routes
Q & A
ANone: While the provided research papers focus heavily on the synthetic utility of 4,5,6-Triaminopyrimidine as a precursor to various heterocyclic compounds, they don't delve into its specific biological targets or downstream effects. More research is needed to elucidate its biological activity profile.
A: * Molecular Formula: C4H7N5 * Molecular Weight: 125.14 g/mol* Spectroscopic Data: The papers discuss characterization using various spectroscopic techniques, including UV-Visible, FTIR, 1H-NMR, 13C-NMR, and HSQC spectroscopy [, , , , , , ]. These techniques provide information about the compound's functional groups, bonding patterns, and overall structure.
ANone: The research papers primarily focus on the compound's reactivity and use in synthetic transformations. Information on its stability under various conditions, compatibility with different materials, or specific applications beyond synthesis is limited in the provided context.
ANone: The provided research does not highlight any catalytic properties of this compound. Its primary role in these studies is as a building block for synthesizing other compounds, such as purines, pyrimidodiazepines, and triazolopyrimidines.
A: While some papers mention structure analysis using NMR [, , ], they don't delve into computational modeling studies like simulations or QSAR models. Further research employing these techniques could provide valuable insights into its reactivity, potential biological targets, and physicochemical properties.
ANone: Information regarding the stability of this compound under various storage conditions or formulation strategies to enhance its stability, solubility, or bioavailability is not extensively discussed in the provided research.
ANone: The provided research primarily focuses on synthetic aspects and does not address SHE regulations related to this compound. Consulting relevant safety data sheets and regulatory guidelines is crucial for handling and using this compound.
ANone: The research papers do not provide data on the absorption, distribution, metabolism, excretion (ADME), or in vivo activity and efficacy of this compound. Further investigations are needed to establish its PK/PD profile.
A: Some studies have investigated the biological activity of derivatives synthesized from this compound. For example, one paper examined the antitumor and antiviral activities of triazolopyrimidines [] synthesized from this compound, while another explored the anticancer, anti-inflammatory, and analgesic properties of various heterocyclic compounds derived from this compound []. These studies suggest the potential of this compound derivatives as lead compounds for drug development.
ANone: The provided research papers do not delve into resistance mechanisms or cross-resistance related to this compound or its derivatives. More research is needed in this area.
ANone: The research primarily focuses on synthetic aspects and does not extensively discuss the toxicology or long-term safety profile of this compound. Standard safety protocols and toxicological studies are essential for handling and evaluating the compound's safety.
ANone: The provided research papers primarily focus on the synthesis and characterization of this compound and its use in preparing various heterocyclic compounds. As a result, they do not provide detailed information on these specific aspects.
A: The studies illustrate the involvement of this compound in different research areas, including organic synthesis, medicinal chemistry, and materials science. For instance, one study explored the use of this compound in developing metal complexes with potential applications in materials science []. This signifies the compound's ability to bridge different disciplines and foster cross-disciplinary research collaborations.
Disclaimer and Information on In-Vitro Research Products
Please be aware that all articles and product information presented on BenchChem are intended solely for informational purposes. The products available for purchase on BenchChem are specifically designed for in-vitro studies, which are conducted outside of living organisms. In-vitro studies, derived from the Latin term "in glass," involve experiments performed in controlled laboratory settings using cells or tissues. It is important to note that these products are not categorized as medicines or drugs, and they have not received approval from the FDA for the prevention, treatment, or cure of any medical condition, ailment, or disease. We must emphasize that any form of bodily introduction of these products into humans or animals is strictly prohibited by law. It is essential to adhere to these guidelines to ensure compliance with legal and ethical standards in research and experimentation.