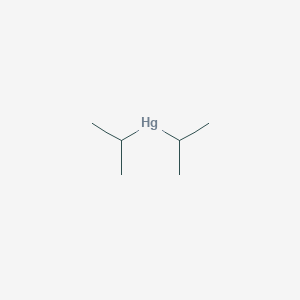
Mercury, diisopropyl-
Overview
Description
Mercury, diisopropyl- is an organomercury compound with the chemical formula ( \text{C}6\text{H}{14}\text{Hg} ). It is a colorless liquid at room temperature and is known for its high toxicity. This compound is primarily used in research settings due to its hazardous nature.
Preparation Methods
Synthetic Routes and Reaction Conditions
Mercury, diisopropyl- can be synthesized through the reaction of mercury(II) chloride with isopropylmagnesium bromide (Grignard reagent) in an anhydrous ether solution. The reaction proceeds as follows: [ \text{HgCl}_2 + 2 \text{C}_3\text{H}_7\text{MgBr} \rightarrow (\text{C}_3\text{H}_7)_2\text{Hg} + 2 \text{MgBrCl} ]
Industrial Production Methods
Due to its high toxicity, mercury, diisopropyl- is not produced on an industrial scale. Its synthesis is typically confined to controlled laboratory environments where strict safety protocols are followed.
Chemical Reactions Analysis
Types of Reactions
Mercury, diisopropyl- undergoes several types of chemical reactions, including:
Oxidation: It can be oxidized to form mercury(II) oxide.
Reduction: It can be reduced to elemental mercury.
Substitution: It can undergo substitution reactions where the isopropyl groups are replaced by other alkyl or aryl groups.
Common Reagents and Conditions
Oxidation: Common oxidizing agents include nitric acid and hydrogen peroxide.
Reduction: Reducing agents such as sodium borohydride or lithium aluminum hydride can be used.
Substitution: Reagents like alkyl halides or aryl halides in the presence of a catalyst can facilitate substitution reactions.
Major Products Formed
Oxidation: Mercury(II) oxide (( \text{HgO} ))
Reduction: Elemental mercury (( \text{Hg} ))
Substitution: Various alkyl or aryl mercury compounds depending on the substituent used.
Scientific Research Applications
Mercury, diisopropyl- is primarily used in scientific research due to its toxic nature. Its applications include:
Chemistry: Used as a reagent in organic synthesis and as a standard in mercury analysis.
Biology: Studied for its effects on biological systems, particularly its toxicological impact.
Medicine: Investigated for its potential use in pharmaceuticals, although its toxicity limits its practical applications.
Industry: Used in small quantities for specialized industrial processes, such as the synthesis of other organomercury compounds.
Mechanism of Action
Mercury, diisopropyl- exerts its effects primarily through the disruption of cellular processes. It binds to thiol groups in proteins, leading to the inhibition of enzyme activity and disruption of cellular function. This binding can cause oxidative stress, leading to cell damage and apoptosis. The compound also interferes with the function of ion channels and receptors, further contributing to its toxic effects.
Comparison with Similar Compounds
Similar Compounds
Dimethylmercury: Another highly toxic organomercury compound with similar properties.
Diethylmercury: Similar in structure but with ethyl groups instead of isopropyl groups.
Diphenylmercury: Contains phenyl groups instead of isopropyl groups.
Uniqueness
Mercury, diisopropyl- is unique due to its specific alkyl groups, which influence its reactivity and toxicity. Compared to dimethylmercury and diethylmercury, it has different steric and electronic properties, affecting its interactions with biological molecules and its overall behavior in chemical reactions.
Biological Activity
Mercury compounds, particularly organomercury compounds such as diisopropyl mercury (DIP), exhibit significant biological activity that can lead to various toxicological effects. This article explores the biological activity of diisopropyl mercury, focusing on its neurotoxicity, oxidative stress induction, and case studies highlighting its impact on human health and the environment.
Diisopropyl mercury is an organomercury compound with the chemical formula . It is a colorless liquid at room temperature and is known for its volatility and ability to cross biological membranes, leading to systemic toxicity.
Mechanisms of Toxicity
Neurotoxicity
Diisopropyl mercury is recognized for its neurotoxic effects. Studies have shown that exposure can lead to cognitive deficits, motor coordination issues, and neurodegeneration. The mechanism involves the disruption of cellular functions, primarily through oxidative stress and apoptosis in neuronal cells. For instance, exposure to inorganic mercury has been linked to increased lipoperoxidation and decreased antioxidant capacity in brain tissues, leading to cell death and functional deficits in the motor cortex .
Oxidative Stress
Mercury compounds induce oxidative stress by generating reactive oxygen species (ROS) that damage cellular components. Research indicates that chronic exposure to inorganic mercury results in significant oxidative damage, characterized by increased nitrite levels and decreased antioxidant defenses . This oxidative stress contributes to neuronal injury and cognitive decline.
Case Study 1: Acute Mercury Poisoning
In a notable case reported in Germany, a 23-year-old male experienced severe neurological symptoms following acute exposure to methylmercury from an unknown source. The patient exhibited dramatic cognitive decline, muscle wasting, and autonomic instability resembling pheochromocytoma. Despite treatment with chelating agents, his condition worsened over time, leading to a persistent vegetative state . This case underscores the severe consequences of mercury exposure on human health.
Case Study 2: School Mercury Incident
A significant incident occurred when a student brought liquid elemental mercury to school, leading to widespread contamination. Over 60 students were exposed, resulting in emergency evacuations and cleanup efforts due to elevated mercury levels in the air . This incident highlights the risks associated with accidental exposure to mercury compounds in educational settings.
Research Findings
Recent studies have provided insights into the biological activity of diisopropyl mercury:
- Neurobehavioral Effects : Chronic exposure studies have demonstrated that even low doses can impair motor functions and induce neurobehavioral changes in animal models .
- Cellular Impact : In vitro studies show that diisopropyl mercury can cause apoptosis in neuronal cell lines through mechanisms involving oxidative stress and mitochondrial dysfunction .
Data Table: Effects of Diisopropyl Mercury Exposure
Properties
IUPAC Name |
di(propan-2-yl)mercury | |
---|---|---|
Source | PubChem | |
URL | https://pubchem.ncbi.nlm.nih.gov | |
Description | Data deposited in or computed by PubChem | |
InChI |
InChI=1S/2C3H7.Hg/c2*1-3-2;/h2*3H,1-2H3; | |
Source | PubChem | |
URL | https://pubchem.ncbi.nlm.nih.gov | |
Description | Data deposited in or computed by PubChem | |
InChI Key |
UVUGOJQWNVFTRT-UHFFFAOYSA-N | |
Source | PubChem | |
URL | https://pubchem.ncbi.nlm.nih.gov | |
Description | Data deposited in or computed by PubChem | |
Canonical SMILES |
CC(C)[Hg]C(C)C | |
Source | PubChem | |
URL | https://pubchem.ncbi.nlm.nih.gov | |
Description | Data deposited in or computed by PubChem | |
Molecular Formula |
C6H14Hg | |
Source | PubChem | |
URL | https://pubchem.ncbi.nlm.nih.gov | |
Description | Data deposited in or computed by PubChem | |
DSSTOX Substance ID |
DTXSID50147914 | |
Record name | Mercury, diisopropyl- | |
Source | EPA DSSTox | |
URL | https://comptox.epa.gov/dashboard/DTXSID50147914 | |
Description | DSSTox provides a high quality public chemistry resource for supporting improved predictive toxicology. | |
Molecular Weight |
286.77 g/mol | |
Source | PubChem | |
URL | https://pubchem.ncbi.nlm.nih.gov | |
Description | Data deposited in or computed by PubChem | |
CAS No. |
1071-39-2 | |
Record name | Bis(1-methylethyl)mercury | |
Source | CAS Common Chemistry | |
URL | https://commonchemistry.cas.org/detail?cas_rn=1071-39-2 | |
Description | CAS Common Chemistry is an open community resource for accessing chemical information. Nearly 500,000 chemical substances from CAS REGISTRY cover areas of community interest, including common and frequently regulated chemicals, and those relevant to high school and undergraduate chemistry classes. This chemical information, curated by our expert scientists, is provided in alignment with our mission as a division of the American Chemical Society. | |
Explanation | The data from CAS Common Chemistry is provided under a CC-BY-NC 4.0 license, unless otherwise stated. | |
Record name | Mercury, diisopropyl- | |
Source | ChemIDplus | |
URL | https://pubchem.ncbi.nlm.nih.gov/substance/?source=chemidplus&sourceid=0001071392 | |
Description | ChemIDplus is a free, web search system that provides access to the structure and nomenclature authority files used for the identification of chemical substances cited in National Library of Medicine (NLM) databases, including the TOXNET system. | |
Record name | Mercury, diisopropyl- | |
Source | EPA DSSTox | |
URL | https://comptox.epa.gov/dashboard/DTXSID50147914 | |
Description | DSSTox provides a high quality public chemistry resource for supporting improved predictive toxicology. | |
Retrosynthesis Analysis
AI-Powered Synthesis Planning: Our tool employs the Template_relevance Pistachio, Template_relevance Bkms_metabolic, Template_relevance Pistachio_ringbreaker, Template_relevance Reaxys, Template_relevance Reaxys_biocatalysis model, leveraging a vast database of chemical reactions to predict feasible synthetic routes.
One-Step Synthesis Focus: Specifically designed for one-step synthesis, it provides concise and direct routes for your target compounds, streamlining the synthesis process.
Accurate Predictions: Utilizing the extensive PISTACHIO, BKMS_METABOLIC, PISTACHIO_RINGBREAKER, REAXYS, REAXYS_BIOCATALYSIS database, our tool offers high-accuracy predictions, reflecting the latest in chemical research and data.
Strategy Settings
Precursor scoring | Relevance Heuristic |
---|---|
Min. plausibility | 0.01 |
Model | Template_relevance |
Template Set | Pistachio/Bkms_metabolic/Pistachio_ringbreaker/Reaxys/Reaxys_biocatalysis |
Top-N result to add to graph | 6 |
Feasible Synthetic Routes
Disclaimer and Information on In-Vitro Research Products
Please be aware that all articles and product information presented on BenchChem are intended solely for informational purposes. The products available for purchase on BenchChem are specifically designed for in-vitro studies, which are conducted outside of living organisms. In-vitro studies, derived from the Latin term "in glass," involve experiments performed in controlled laboratory settings using cells or tissues. It is important to note that these products are not categorized as medicines or drugs, and they have not received approval from the FDA for the prevention, treatment, or cure of any medical condition, ailment, or disease. We must emphasize that any form of bodily introduction of these products into humans or animals is strictly prohibited by law. It is essential to adhere to these guidelines to ensure compliance with legal and ethical standards in research and experimentation.