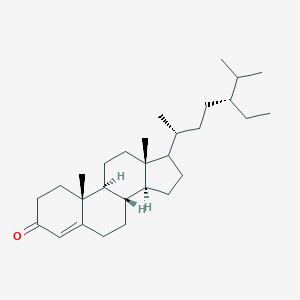
Sitostenone
Overview
Description
Sitostenone, a derivative of β-sitosterol, is a bioactive compound found in plants and has been studied for its potential therapeutic effects. It is a steroid-like compound that has shown promise in promoting glucose uptake and improving insulin sensitivity in hepatic cells, which could be beneficial for diabetic management . This compound and its related compounds, such as β-sitosterol, are abundant in lipid-rich plant foods and have been associated with various biological actions, including anti-inflammatory, lipid-lowering, and anti-cancer activities .
Synthesis Analysis
The synthesis of this compound and its derivatives involves various chemical reactions and processes. For instance, β-sitosterol has been synthesized by esterification with poly(ethylene glycol) to create hydrophilic derivatives, which have different solubility properties . Additionally, sitosterol can be transformed by bacteria, which can lead to the splitting of the side chain in the sterol molecule, a necessary step for the synthesis of steroid hormones . The synthesis of β-sitosterol labeled with carbon-14 has also been reported, which is important for tracing and studying the biological significance of this compound .
Molecular Structure Analysis
β-Sitosterol, the precursor of this compound, has a molecular formula of C29H50O and is structurally similar to cholesterol . This similarity allows it to integrate into cell membranes and potentially influence various biological processes. The molecular structure of this compound itself is not detailed in the provided papers, but it is implied that it retains the steroidal backbone characteristic of β-sitosterol.
Chemical Reactions Analysis
Sitosterol can undergo various chemical reactions, including esterification and oxidation. The esterification of β-sitosterol with carboxylic functionality has been used to create hydrophilic derivatives . Oxidation reactions have been employed to modify the sitosterol molecule, as seen in the synthesis of sitosterol-14C, where an enol-lactone of the ketoacid of sitosterol is condensed with phenyl acetate . Additionally, the transformation of sitosterol by bacteria can result in the formation of different compounds, such as sitost-4-en-3-one and androst-4-ene-3,17-dione, which are intermediates in the production of steroid hormones .
Physical and Chemical Properties Analysis
The physical and chemical properties of this compound are closely related to those of β-sitosterol. β-Sitosterol is described as a white powdery substance , and its derivatives' solubility can be modified through chemical synthesis, as demonstrated by the creation of hydrophilic β-sitosterol derivatives . The biosynthesis of sitosterol in plants involves the incorporation of deuterium into newly biosynthesized sterols, indicating the presence of specific isotopes in the sterol side chain . The solubility and stability of sitosterol derivatives are important for their potential use in pharmaceutical applications, as seen in the synthesis of a curcumin-β-sitosterol conjugate, which exhibited good cytotoxicity against cancer cells .
Scientific Research Applications
Anti-Diabetic Effects : Sitostenone isolated from Rosa laevigata fruits has shown promising ability to promote glucose uptake and improve insulin resistance in hepatic cells. It activates proteins involved in the insulin signal transduction pathway and provokes glucose uptake through PPAR-γ and AMPK activation, facilitating the regulation of glucose transporters (Kumar, Lin, Tseng, & Wang, 2021).
Platelet Hyperreactivity and Bleeding Abnormality : In a study on sitosterolemia, a condition caused by mutations leading to sterol accumulation, this compound was implicated in the hyperactivation of platelets. This hyperreactivity contributes to macrothrombocytopenia and bleeding abnormalities, demonstrating its significant role in platelet physiology (Kanaji, Kanaji, Montgomery, Patel, & Newman, 2013).
Antimicrobial and Cytotoxic Properties : this compound has shown significant antimicrobial and cytotoxic properties. It was isolated from Annona montana and demonstrated potent activity against Bacillus subtilis and Micrococcus luteus, as well as in cytotoxicity assays (Wu, Jong, Tien, Kuoh, Furukawa, & Lee, 1987).
Anti-Inflammatory Effects : β-sitostenone was identified as an active component in Terminalia phanerophlebia, showing significant anti-inflammatory effects by inhibiting the cyclooxygenase enzyme COX-2. This discovery is relevant for its use in traditional medicine for treating inflammation and other conditions (Nair, Aremu, & Staden, 2012).
Phytosterol Stability : Research on the stabilization of phytosterols under high-temperature conditions identified β-sitostenone as a key compound. The study evaluated the effectiveness of various antioxidants in protecting phytosterols like β-sitostenone from degradation, highlighting its importance in food chemistry (Kmiecik, Korczak, Rudzińska, Gramza-Michałowska, Hęś, & Kobus‐Cisowska, 2015).
Geographical Variation in Bioactive Constituents : A study on Prunus africana across Africa found geographical variation in the concentration of bioactive constituents, including β-sitostenone. This variation was associated with environmental and genetic factors, underlining the importance of geographic and phylogenetic considerations in the study of plant-based compounds (Kadu et al., 2012).
Mechanism of Action
Target of Action
Stigmast-4-en-3-one, also known as Sitostenone, primarily targets the E2F1 protein . E2F1 is a transcription factor that plays a crucial role in the control of the cell cycle and the action of tumor suppressor proteins. It is also associated with apoptosis .
Mode of Action
This compound interacts with its target, E2F1, by reducing its expression . This reduction in E2F1 expression leads to a decrease in the viability of liver cancer cells and induces apoptosis and ferroptosis .
Biochemical Pathways
The reduction of E2F1 by this compound affects several biochemical pathways. It increases the expression of Bax, a pro-apoptotic protein, and reduces the expression of GPX4, a key regulator of ferroptosis . Additionally, it enhances the cleavage of PARP, a protein involved in DNA repair and programmed cell death .
Pharmacokinetics
It is known that this compound is produced by the biotransformation of β-sitosterol using rhodococcus cells . The maximum formation of this compound is achieved in the presence of n-hexadecane and palmitic acid .
Result of Action
The action of this compound results in decreased viability and induced apoptosis and ferroptosis in liver cancer cells . It also increases cellular malondialdehyde (MDA) and lipid peroxidation levels . These changes contribute to the death of cancer cells and potentially slow the progression of the disease .
Action Environment
The action of this compound can be influenced by various environmental factors. For instance, the presence of n-hexadecane and palmitic acid enhances the formation of this compound . .
Safety and Hazards
Biochemical Analysis
Biochemical Properties
Stigmast-4-en-3-one plays a significant role in biochemical reactions. It is produced by the biotransformation of β-sitosterol using Rhodococcus actinobacteria . This process involves the oxidation of β-sitosterol, which is facilitated by the cholesterol oxidase reaction induced by palmitic acid . The maximum formation of Stigmast-4-en-3-one is achieved using an additional growth substrate n-hexadecane .
Cellular Effects
Stigmast-4-en-3-one has been found to have significant effects on various types of cells. For instance, it has been reported to decrease the viability and induce apoptosis and ferroptosis in liver cancer cells . This compound influences cell function by reducing E2F1, a transcription factor involved in cell cycle regulation .
Molecular Mechanism
The molecular mechanism of Stigmast-4-en-3-one involves its interaction with various biomolecules. It has been found to reduce the expression of E2F1 and upregulate p53 at both the mRNA and protein levels . This leads to changes in gene expression, which in turn influences cell function .
Temporal Effects in Laboratory Settings
In laboratory settings, the effects of Stigmast-4-en-3-one have been observed to change over time. The biocatalytic oxidation of β-sitosterol to Stigmast-4-en-3-one was optimized to decrease the bioconversion process duration from 7 to 5 days . This indicates the compound’s stability and its long-term effects on cellular function.
Dosage Effects in Animal Models
The effects of Stigmast-4-en-3-one vary with different dosages in animal models. For instance, it has been found to exhibit significant antiplasmodial activity against Plasmodium falciparum 3D7, with IC50 values of 43.54 μg/mL . This suggests that the compound may have potential therapeutic applications in the treatment of malaria.
Metabolic Pathways
Stigmast-4-en-3-one is involved in the metabolic pathway of β-sitosterol biotransformation . This process involves the oxidation of β-sitosterol, facilitated by the cholesterol oxidase reaction induced by palmitic acid . The compound interacts with enzymes such as cholesterol oxidase and cofactors like palmitic acid in this pathway .
properties
IUPAC Name |
(8S,9S,10R,13R,14S,17R)-17-[(2R,5R)-5-ethyl-6-methylheptan-2-yl]-10,13-dimethyl-1,2,6,7,8,9,11,12,14,15,16,17-dodecahydrocyclopenta[a]phenanthren-3-one | |
---|---|---|
Source | PubChem | |
URL | https://pubchem.ncbi.nlm.nih.gov | |
Description | Data deposited in or computed by PubChem | |
InChI |
InChI=1S/C29H48O/c1-7-21(19(2)3)9-8-20(4)25-12-13-26-24-11-10-22-18-23(30)14-16-28(22,5)27(24)15-17-29(25,26)6/h18-21,24-27H,7-17H2,1-6H3/t20-,21-,24+,25-,26+,27+,28+,29-/m1/s1 | |
Source | PubChem | |
URL | https://pubchem.ncbi.nlm.nih.gov | |
Description | Data deposited in or computed by PubChem | |
InChI Key |
RUVUHIUYGJBLGI-XJZKHKOHSA-N | |
Source | PubChem | |
URL | https://pubchem.ncbi.nlm.nih.gov | |
Description | Data deposited in or computed by PubChem | |
Canonical SMILES |
CCC(CCC(C)C1CCC2C1(CCC3C2CCC4=CC(=O)CCC34C)C)C(C)C | |
Source | PubChem | |
URL | https://pubchem.ncbi.nlm.nih.gov | |
Description | Data deposited in or computed by PubChem | |
Isomeric SMILES |
CC[C@H](CC[C@@H](C)[C@H]1CC[C@@H]2[C@@]1(CC[C@H]3[C@H]2CCC4=CC(=O)CC[C@]34C)C)C(C)C | |
Source | PubChem | |
URL | https://pubchem.ncbi.nlm.nih.gov | |
Description | Data deposited in or computed by PubChem | |
Molecular Formula |
C29H48O | |
Source | PubChem | |
URL | https://pubchem.ncbi.nlm.nih.gov | |
Description | Data deposited in or computed by PubChem | |
DSSTOX Substance ID |
DTXSID50862519 | |
Record name | Stigmast-4-en-3-one | |
Source | EPA DSSTox | |
URL | https://comptox.epa.gov/dashboard/DTXSID50862519 | |
Description | DSSTox provides a high quality public chemistry resource for supporting improved predictive toxicology. | |
Molecular Weight |
412.7 g/mol | |
Source | PubChem | |
URL | https://pubchem.ncbi.nlm.nih.gov | |
Description | Data deposited in or computed by PubChem | |
CAS RN |
1058-61-3, 67392-96-5 | |
Record name | Stigmast-4-en-3-one | |
Source | CAS Common Chemistry | |
URL | https://commonchemistry.cas.org/detail?cas_rn=1058-61-3 | |
Description | CAS Common Chemistry is an open community resource for accessing chemical information. Nearly 500,000 chemical substances from CAS REGISTRY cover areas of community interest, including common and frequently regulated chemicals, and those relevant to high school and undergraduate chemistry classes. This chemical information, curated by our expert scientists, is provided in alignment with our mission as a division of the American Chemical Society. | |
Explanation | The data from CAS Common Chemistry is provided under a CC-BY-NC 4.0 license, unless otherwise stated. | |
Record name | Stigmast-4-en-3-one | |
Source | ChemIDplus | |
URL | https://pubchem.ncbi.nlm.nih.gov/substance/?source=chemidplus&sourceid=0001058613 | |
Description | ChemIDplus is a free, web search system that provides access to the structure and nomenclature authority files used for the identification of chemical substances cited in National Library of Medicine (NLM) databases, including the TOXNET system. | |
Record name | 24-Ethyl-4-cholesten-3-one | |
Source | ChemIDplus | |
URL | https://pubchem.ncbi.nlm.nih.gov/substance/?source=chemidplus&sourceid=0067392965 | |
Description | ChemIDplus is a free, web search system that provides access to the structure and nomenclature authority files used for the identification of chemical substances cited in National Library of Medicine (NLM) databases, including the TOXNET system. | |
Record name | Stigmast-4-en-3-one | |
Source | EPA DSSTox | |
URL | https://comptox.epa.gov/dashboard/DTXSID50862519 | |
Description | DSSTox provides a high quality public chemistry resource for supporting improved predictive toxicology. | |
Retrosynthesis Analysis
AI-Powered Synthesis Planning: Our tool employs the Template_relevance Pistachio, Template_relevance Bkms_metabolic, Template_relevance Pistachio_ringbreaker, Template_relevance Reaxys, Template_relevance Reaxys_biocatalysis model, leveraging a vast database of chemical reactions to predict feasible synthetic routes.
One-Step Synthesis Focus: Specifically designed for one-step synthesis, it provides concise and direct routes for your target compounds, streamlining the synthesis process.
Accurate Predictions: Utilizing the extensive PISTACHIO, BKMS_METABOLIC, PISTACHIO_RINGBREAKER, REAXYS, REAXYS_BIOCATALYSIS database, our tool offers high-accuracy predictions, reflecting the latest in chemical research and data.
Strategy Settings
Precursor scoring | Relevance Heuristic |
---|---|
Min. plausibility | 0.01 |
Model | Template_relevance |
Template Set | Pistachio/Bkms_metabolic/Pistachio_ringbreaker/Reaxys/Reaxys_biocatalysis |
Top-N result to add to graph | 6 |
Feasible Synthetic Routes
Q & A
Q1: What is the molecular formula and weight of Sitostenone?
A1: this compound has the molecular formula C29H48O and a molecular weight of 412.69 g/mol.
Q2: What spectroscopic data is available for this compound?
A2: Structural characterization of this compound commonly involves techniques like Nuclear Magnetic Resonance (NMR) spectroscopy (both 1H and 13C), Infrared (IR) spectroscopy, and Mass Spectrometry (MS). These methods help determine the compound's structure and confirm its identity. [, , , , , ]
Q3: Is this compound found naturally? If so, where?
A3: Yes, this compound is a naturally occurring steroid compound found in various plants. It has been isolated from the bark of Cryptomeria japonica [], the fruits of Terminalia catappa L. [], and the twigs of Millettia utilis Dunn. [], among other sources.
Q4: What are some potential biological activities of this compound?
A4: Research suggests that this compound might possess various biological activities, including:
- Anti-melanogenic properties: Studies indicate that this compound can inhibit tyrosinase activity and reduce melanin content in B16F10 cells, suggesting potential in cosmetic skin whitening. []
- α-glucosidase inhibitory activity: this compound has shown inhibitory activity against α-glucosidase, an enzyme involved in carbohydrate metabolism, suggesting potential for managing type II diabetes mellitus. []
- Antibacterial activity: this compound, isolated from Cryptomeria japonica bark, displayed antibacterial activity, suggesting its potential as a natural bactericide. []
- Potential SARS-CoV-2 MPro inhibitor: Molecular docking studies suggest this compound could be a potential inhibitor of the SARS-CoV-2 main protease (MPro), a crucial target for COVID-19 treatment. []
Q5: How does this compound exert its α-glucosidase inhibitory effect?
A5: Molecular docking studies revealed that this compound exhibits a strong binding affinity to the α-glucosidase enzyme, specifically from Saccharomyces cerevisiae. The binding energy (ΔG) was calculated to be -10.61 kcal/mol with an inhibition constant (Ki) of 0.02 µM. The this compound molecule interacts with 18 amino acid residues within the enzyme's active site, potentially hindering its catalytic activity and thus inhibiting carbohydrate breakdown. []
Q6: What are the potential applications of this compound in drug development?
A6: this compound's diverse biological activities make it a promising candidate for drug development, particularly in the following areas:
Q7: What is known about the stability of this compound?
A7: While detailed information on the stability of this compound under various conditions is limited in the provided research, one study suggests that the extraction method may impact its stability. A Soxhlet extraction of Gongronema latifolium, which contains this compound, resulted in moderate antidiabetic activity. The authors propose that this extraction method might degrade heat-liable compounds, potentially impacting this compound's stability and activity. []
Q8: What is known about the safety and toxicology of this compound?
A8: The provided research does not include comprehensive data on the toxicity profile and long-term effects of this compound. Further investigation is crucial to establish its safety for potential therapeutic applications.
Q9: What analytical techniques are used to identify and quantify this compound?
A9: Various analytical techniques are employed to identify and quantify this compound, including:
- Gas Chromatography-Mass Spectrometry (GC-MS): This technique is commonly used to separate and identify volatile and semi-volatile compounds, like this compound, in complex mixtures based on their mass-to-charge ratio. [, , , ]
- High-Performance Liquid Chromatography (HPLC): HPLC is a versatile technique used to separate, identify, and quantify this compound in various matrices, particularly when combined with detection methods like UV-Vis or mass spectrometry. [, ]
- Thin Layer Chromatography (TLC): This simple and cost-effective technique is employed for the initial screening and separation of this compound from other compounds in plant extracts. [, ]
Disclaimer and Information on In-Vitro Research Products
Please be aware that all articles and product information presented on BenchChem are intended solely for informational purposes. The products available for purchase on BenchChem are specifically designed for in-vitro studies, which are conducted outside of living organisms. In-vitro studies, derived from the Latin term "in glass," involve experiments performed in controlled laboratory settings using cells or tissues. It is important to note that these products are not categorized as medicines or drugs, and they have not received approval from the FDA for the prevention, treatment, or cure of any medical condition, ailment, or disease. We must emphasize that any form of bodily introduction of these products into humans or animals is strictly prohibited by law. It is essential to adhere to these guidelines to ensure compliance with legal and ethical standards in research and experimentation.