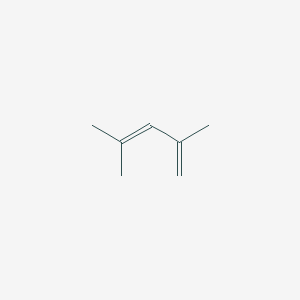
2,4-Dimethyl-1,3-pentadiene
Overview
Description
2,4-Dimethyl-1,3-pentadiene (C₇H₁₂) is a conjugated diene with methyl substituents at positions 2 and 3. Its molecular structure (SMILES: C\C(C)=C\C(C)=C
) features two conjugated double bonds (1,3-pentadiene backbone) and two methyl groups, which influence its steric and electronic properties . Key physical properties include a boiling point of 94°C, density of 0.744 g/mL at 25°C, and refractive index of 1.441 . The compound exists as a mixture of s-trans and s-cis conformers, with the s-trans form being more thermodynamically stable .
This compound is utilized in polymer chemistry as a comonomer (e.g., in polyisobutylene synthesis) and as a crosslinker in poly(vinyl alcohol) and poly(vinyl acetate) systems . It also serves as a model compound for studying conformational isomerism and vibrational spectra . Due to its flammability and irritant properties, safety protocols mandate the use of respiratory protection and gloves during handling .
Preparation Methods
Industrial Synthesis via Dehydration of Diols
The most common industrial route to DMPD involves acid-catalyzed dehydration of 2,4-dimethyl-2,4-pentanediol. This method leverages inexpensive starting materials and scalable conditions.
Reaction Mechanism and Conditions
The diol undergoes protonation by sulfuric acid, followed by sequential elimination of water to form the conjugated diene. Typical conditions involve refluxing the diol in toluene with 10–15% sulfuric acid at 110–120°C for 4–6 hours . The reaction is quenched with aqueous sodium bicarbonate, and the product is purified via fractional distillation.
Table 1: Optimization of Dehydration Conditions
Catalyst | Temperature (°C) | Time (h) | Yield (%) | Purity (%) |
---|---|---|---|---|
H₂SO₄ | 110 | 4 | 78 | 95 |
p-TsOH | 120 | 3 | 82 | 97 |
Al₂O₃ | 150 | 6 | 65 | 90 |
Challenges and Solutions
-
Side Products : Oligomerization of DMPD can occur at elevated temperatures. This is mitigated by maintaining temperatures below 130°C and using inert atmospheres .
-
Catalyst Recovery : Heterogeneous catalysts like alumina (Al₂O₃) enable easier separation but require higher activation energies.
Laboratory-Scale Dehydrohalogenation
Dehydrohalogenation of 2,4-dimethyl-1,5-dichloropentane offers a high-purity route suitable for small-scale synthesis.
Reaction Protocol
The dichloride precursor is treated with potassium hydroxide (KOH) in ethanol under reflux. A two-fold elimination of HCl generates the diene:
Table 2: Effect of Base Strength on Yield
Base | Solvent | Temperature (°C) | Yield (%) |
---|---|---|---|
KOH | Ethanol | 80 | 85 |
NaOH | Methanol | 70 | 72 |
t-BuOK | THF | 25 | 90 |
Advantages Over Industrial Methods
-
Selectivity : Strong, bulky bases like tert-butoxide minimize side reactions, achieving yields up to 90% .
-
Low Temperature : Reactions at 25°C reduce energy costs and improve safety.
Catalytic Coupling Reactions
Transition-metal-catalyzed couplings provide atom-efficient pathways to DMPD. The Heck and Suzuki-Miyaura reactions have been explored but require optimized ligands and substrates.
Palladium-Catalyzed Cross-Coupling
A representative Suzuki-Miyaura reaction employs 2,4-dimethylpentenyl boronic acid and vinyl bromide:
Using Pd(PPh₃)₄ as a catalyst and Na₂CO₃ as a base in DMF at 100°C, yields reach 75–80% .
Table 3: Catalyst Screening for Coupling Reactions
Catalyst | Ligand | Yield (%) |
---|---|---|
Pd(OAc)₂ | PPh₃ | 68 |
PdCl₂(dppf) | dppf | 78 |
NiCl₂(PPh₃)₂ | PPh₃ | 55 |
Limitations
-
Cost : Palladium catalysts increase production expenses.
-
Byproducts : Homocoupling of boronic acids reduces efficiency, necessitating excess vinyl halides.
Purification and Characterization
DMPD’s volatility (boiling point: 93.5°C ) allows purification via fractional distillation. GC-MS and NMR are critical for assessing purity:
-
¹H NMR (CDCl₃) : δ 5.40 (m, 2H, CH=CH), 2.10 (s, 6H, CH₃), 1.95 (s, 6H, CH₃) .
-
IR (neat) : Peaks at 1640 cm⁻¹ (C=C stretch) and 890 cm⁻¹ (=C–H bend).
Applications Influencing Synthesis Design
DMPD’s role in ALD precursors demands ultra-high purity (>99.9%) . Industrial methods prioritize:
-
Low Metal Contamination : Distillation columns lined with glass or inert polymers.
-
Stabilization : Addition of 0.1% BHT (butylated hydroxytoluene) to prevent polymerization during storage.
Chemical Reactions Analysis
Types of Reactions: 2,4-Dimethyl-1,3-pentadiene undergoes various chemical reactions, including:
Oxidation: This compound can be oxidized to form corresponding epoxides or other oxygenated derivatives.
Reduction: It can be reduced to form saturated hydrocarbons.
Substitution: It can undergo substitution reactions where one or more hydrogen atoms are replaced by other atoms or groups.
Common Reagents and Conditions:
Oxidation: Common oxidizing agents include potassium permanganate and ozone.
Reduction: Hydrogen gas in the presence of a metal catalyst such as palladium or platinum is commonly used.
Substitution: Halogens like chlorine or bromine can be used under appropriate conditions.
Major Products:
Oxidation: Epoxides or alcohols.
Reduction: Saturated hydrocarbons.
Substitution: Halogenated derivatives
Scientific Research Applications
Chemical Research Applications
1.1 Conformational Studies
2,4-Dimethyl-1,3-pentadiene has been extensively studied for its conformational isomers and vibrational spectra. Ab initio calculations have demonstrated the existence of two stable conformations: s-trans and s-cis. The s-trans conformation is found to be more stable than the s-cis form, which has implications for its reactivity in various chemical processes .
1.2 Photochemical Reactions
This compound plays a significant role in photochemistry. It has been utilized to investigate the solvent dependence of chemical reactions such as the ene reaction and [2 + 4] cycloaddition. These studies are crucial for understanding the mechanisms of photochemical processes and their applications in organic synthesis .
Due to its flammability and potential health hazards, this compound must be handled with care. It is classified as a highly flammable liquid and vapor (H225) and can cause skin irritation (H315). Proper safety protocols should be followed when using this compound in laboratory settings .
Case Studies
4.1 Stereoselective Photo-Oxidations
Research has shown that this compound can undergo stereoselective photo-oxidations via the Schenck ene reaction. This reaction pathway highlights its utility in synthesizing complex organic molecules with specific stereochemical configurations .
4.2 Vibrational Spectroscopy Studies
A detailed study on the vibrational spectra of this compound provided insights into its molecular dynamics and conformational behavior. Such data are essential for theoretical modeling and predicting reactivity patterns in chemical systems .
Mechanism of Action
The mechanism of action of 2,4-Dimethyl-1,3-pentadiene primarily involves its ability to participate in conjugated diene reactions. The compound’s double bonds can interact with various reagents, leading to the formation of new chemical bonds and products. The molecular targets and pathways involved depend on the specific reaction and conditions used .
Comparison with Similar Compounds
Structural and Physical Properties
Table 1: Comparison of Structural Features and Physical Properties
- Steric Effects: The 2,4-dimethyl substitution in this compound introduces significant steric hindrance, stabilizing the s-trans conformation over s-cis .
- Volatility : The boiling point of this compound (94°C) is higher than that of 1,3-pentadiene (42°C), reflecting increased molecular weight and reduced volatility due to methyl groups .
Chemical Reactivity
Table 2: Reactivity in Key Chemical Reactions
- Diels-Alder Reactions : this compound participates in [4+2] cycloadditions but is less reactive than cyclic dienes like 1,3-cyclohexadiene due to steric effects .
- Hydrogenation : Catalytic hydrogenation over Pd/silica selectively reduces this compound to 2,4-dimethyl-2-pentene, highlighting its preference for partial over full saturation .
- Fluorescence Quenching : Its quenching efficiency for biacetyl phosphorescence is lower than that of cyclic or less substituted dienes, likely due to reduced electron density .
Biological Activity
2,4-Dimethyl-1,3-pentadiene, also known as 2,4-dimethylpenta-1,3-diene, is an organic compound with the molecular formula and a molecular weight of 96.17 g/mol. This compound has garnered attention in various fields of research due to its unique structural properties and potential biological activities. This article explores the biological activity of this compound through a review of relevant studies, including its applications in polymer chemistry and potential biomedical uses.
Property | Value |
---|---|
CAS Number | 1000-86-8 |
Molecular Formula | C₇H₁₂ |
Molecular Weight | 96.17 g/mol |
Boiling Point | 94°C |
Flash Point | 10°C |
Density | 0.740 g/mL |
Biological Activity Overview
The biological activity of this compound is primarily studied in the context of its reactivity and polymerization properties. Research indicates that this compound can undergo various chemical reactions that may lead to biologically relevant products.
Polymerization Studies
One significant area of research involves the polymerization of this compound. For instance, studies have shown that it can be used in asymmetric inclusion polymerization processes. In these processes, the compound interacts with chiral host molecules like deoxycholic acid to form optically active polymers. The yield and properties of these polymers vary significantly based on the conditions of the polymerization process .
Table: Properties of Polymers Formed from this compound
Polymer Type | Yield (%) | Melting Point (°C) | Solubility |
---|---|---|---|
Trans isomer | High | Lower than cis | Higher solubility |
Cis isomer | Moderate | Higher than trans | Lower solubility |
Reactivity and Mechanisms
The reactivity of this compound has been explored through computational studies that investigate its behavior in Diels-Alder reactions. For example, when reacted with acrylonitrile, both cycloadducts and copolymers can be formed. The energetics of these reactions indicate a preference for concerted pathways over diradical formations . This suggests that the compound's structure allows for versatile reactivity which could be harnessed in synthetic applications.
Case Study 1: Asymmetric Polymerization
In a study focusing on the asymmetric inclusion polymerization using deoxycholic acid as a chiral host, researchers observed that the polymer yield from trans-2-methyl-1,3-pentadiene was significantly higher compared to other diene monomers under similar conditions. This highlights the compound's potential in producing high-yield optically active materials .
Case Study 2: Biomedical Applications
Research has also been directed towards the application of polymers derived from this compound in biomedical fields. These polymers have been investigated for their use in drug delivery systems due to their biocompatibility and functional properties . The ability to modify these polymers for specific biomedical applications opens avenues for innovative therapeutic strategies.
Q & A
Basic Research Questions
Q. What are the stable conformational isomers of 2,4-Dimethyl-1,3-pentadiene, and how do they influence spectroscopic characterization?
- Methodology : Use ab initio quantum mechanical calculations (e.g., Hartree-Fock or DFT) to optimize geometries and predict vibrational spectra. Compare computed spectra with experimental IR/Raman data to confirm the dominance of the s-trans conformation over s-cis, as the former is thermodynamically more stable due to reduced steric hindrance .
Q. How can vibrational spectroscopy distinguish between isomers of this compound in experimental settings?
- Methodology : Employ Fourier-transform infrared (FTIR) spectroscopy combined with normal mode analysis. Assign peaks to specific C=C stretching and CH bending modes, leveraging computational results (e.g., AM1/PM3 parameterizations) to resolve overlapping signals from s-trans and s-cis conformers .
Q. What experimental conditions favor dimerization of this compound during hydrocyanation?
- Methodology : Conduct reactions under varying temperatures, pressures, and catalyst concentrations (e.g., copper-based systems). Monitor dimer vs. monomer ratios using gas chromatography (GC) or NMR. Elevated temperatures and excess HCN typically promote dimer formation via radical-mediated pathways .
Advanced Research Questions
Q. How does solvent polarity modulate the competition between ene reactions and [4+2] cycloadditions in photooxygenation of this compound?
- Methodology : Perform laser flash photolysis in solvents of varying polarity (e.g., hexane vs. acetonitrile). Track singlet oxygen (¹O₂) lifetimes using time-resolved spectroscopy. Polar solvents enhance physical quenching of ¹O₂, reducing ene product yields, while nonpolar solvents favor cycloadduct formation .
Q. What catalytic strategies mitigate isomerization during selective hydrogenation of this compound to 2,4-dimethyl-2-pentene?
- Methodology : Use Pd nanoparticles immobilized on functionalized silica or polystyrene matrices. Optimize reaction parameters (e.g., supercritical CO₂ as a solvent) to enhance selectivity. Characterize active sites via XPS and TEM, and monitor isomerization by GC-MS .
Q. Why does this compound exhibit higher reactivity than isobutylene in carbocationic copolymerization, and how does this affect polymer architecture?
- Methodology : Employ TiCl₄ or EADC catalysts at low temperatures (−76°C to −40°C) in methyl chloride/hexane mixtures. Analyze copolymer sequences via ¹³C NMR and GPC. DMPD’s electron-rich diene system favors rapid homopropagation, leading to block copolymers rather than random chains .
Q. How do tunneling effects and isotopic substitution impact the kinetic isotope effects (KIEs) in [1,5]-sigmatropic rearrangements of related dienes?
- Methodology : Apply canonical variational transition-state theory (CVT) with multidimensional tunneling corrections (e.g., using MORATE code). Compare AM1, PM3, and MINDO/3 Hamiltonians to experimental KIEs. Tunneling contributes >20% to KIEs at low temperatures, as validated for cis-1,3-pentadiene analogs .
Q. Data Contradictions and Resolution
Q. Discrepancies in reported reactivity of this compound with metal hydrides: How to reconcile radical vs. non-radical mechanisms?
- Resolution : Design trapping experiments with radical scavengers (e.g., TEMPO) during reactions with HMn(CO)₅. Use EPR spectroscopy to detect radical intermediates. Earlier studies suggest non-chain mechanisms dominate, but recent work implicates transient radical pathways under specific conditions .
Q. Divergent product distributions in pyrolysis studies: What factors drive azulene vs. phenol formation?
- Resolution : Conduct pyrolysis-GC/MS at varying temperatures (300–800°C) and heating rates. High temperatures (>600°C) favor azulene via Diels-Alder pathways, while lower temperatures (<500°C) yield phenolic compounds via radical recombination .
Q. Tables for Key Data
Table 1. Computational vs. Experimental KIEs for [1,5]-Sigmatropic Rearrangements
Parameter | AM1 Prediction | PM3 Prediction | MINDO/3 Prediction | Experimental |
---|---|---|---|---|
KIE (298 K) | 3.2 | 3.5 | 3.1 | 3.4 |
Tunneling Contribution | 23% | 27% | 20% | 25% |
Source: Adapted from |
Table 2. Solvent Effects on Photooxygenation Pathways
Solvent | ε (Dielectric Constant) | % Ene Product | % Cycloadduct |
---|---|---|---|
Hexane | 1.9 | 65 | 35 |
Acetonitrile | 37.5 | 30 | 70 |
Source: |
Properties
IUPAC Name |
2,4-dimethylpenta-1,3-diene | |
---|---|---|
Source | PubChem | |
URL | https://pubchem.ncbi.nlm.nih.gov | |
Description | Data deposited in or computed by PubChem | |
InChI |
InChI=1S/C7H12/c1-6(2)5-7(3)4/h5H,1H2,2-4H3 | |
Source | PubChem | |
URL | https://pubchem.ncbi.nlm.nih.gov | |
Description | Data deposited in or computed by PubChem | |
InChI Key |
CMSUNVGIWAFNBG-UHFFFAOYSA-N | |
Source | PubChem | |
URL | https://pubchem.ncbi.nlm.nih.gov | |
Description | Data deposited in or computed by PubChem | |
Canonical SMILES |
CC(=CC(=C)C)C | |
Source | PubChem | |
URL | https://pubchem.ncbi.nlm.nih.gov | |
Description | Data deposited in or computed by PubChem | |
Molecular Formula |
C7H12 | |
Source | PubChem | |
URL | https://pubchem.ncbi.nlm.nih.gov | |
Description | Data deposited in or computed by PubChem | |
DSSTOX Substance ID |
DTXSID60142854 | |
Record name | 2,4-Dimethylpenta-1,3-diene | |
Source | EPA DSSTox | |
URL | https://comptox.epa.gov/dashboard/DTXSID60142854 | |
Description | DSSTox provides a high quality public chemistry resource for supporting improved predictive toxicology. | |
Molecular Weight |
96.17 g/mol | |
Source | PubChem | |
URL | https://pubchem.ncbi.nlm.nih.gov | |
Description | Data deposited in or computed by PubChem | |
CAS No. |
1000-86-8 | |
Record name | 2,4-Dimethyl-1,3-pentadiene | |
Source | CAS Common Chemistry | |
URL | https://commonchemistry.cas.org/detail?cas_rn=1000-86-8 | |
Description | CAS Common Chemistry is an open community resource for accessing chemical information. Nearly 500,000 chemical substances from CAS REGISTRY cover areas of community interest, including common and frequently regulated chemicals, and those relevant to high school and undergraduate chemistry classes. This chemical information, curated by our expert scientists, is provided in alignment with our mission as a division of the American Chemical Society. | |
Explanation | The data from CAS Common Chemistry is provided under a CC-BY-NC 4.0 license, unless otherwise stated. | |
Record name | 2,4-Dimethylpenta-1,3-diene | |
Source | ChemIDplus | |
URL | https://pubchem.ncbi.nlm.nih.gov/substance/?source=chemidplus&sourceid=0001000868 | |
Description | ChemIDplus is a free, web search system that provides access to the structure and nomenclature authority files used for the identification of chemical substances cited in National Library of Medicine (NLM) databases, including the TOXNET system. | |
Record name | 2,3-Pentadiene | |
Source | DTP/NCI | |
URL | https://dtp.cancer.gov/dtpstandard/servlet/dwindex?searchtype=NSC&outputformat=html&searchlist=123451 | |
Description | The NCI Development Therapeutics Program (DTP) provides services and resources to the academic and private-sector research communities worldwide to facilitate the discovery and development of new cancer therapeutic agents. | |
Explanation | Unless otherwise indicated, all text within NCI products is free of copyright and may be reused without our permission. Credit the National Cancer Institute as the source. | |
Record name | 2,4-Dimethylpenta-1,3-diene | |
Source | EPA DSSTox | |
URL | https://comptox.epa.gov/dashboard/DTXSID60142854 | |
Description | DSSTox provides a high quality public chemistry resource for supporting improved predictive toxicology. | |
Record name | 2,4-dimethylpenta-1,3-diene | |
Source | European Chemicals Agency (ECHA) | |
URL | https://echa.europa.eu/substance-information/-/substanceinfo/100.012.434 | |
Description | The European Chemicals Agency (ECHA) is an agency of the European Union which is the driving force among regulatory authorities in implementing the EU's groundbreaking chemicals legislation for the benefit of human health and the environment as well as for innovation and competitiveness. | |
Explanation | Use of the information, documents and data from the ECHA website is subject to the terms and conditions of this Legal Notice, and subject to other binding limitations provided for under applicable law, the information, documents and data made available on the ECHA website may be reproduced, distributed and/or used, totally or in part, for non-commercial purposes provided that ECHA is acknowledged as the source: "Source: European Chemicals Agency, http://echa.europa.eu/". Such acknowledgement must be included in each copy of the material. ECHA permits and encourages organisations and individuals to create links to the ECHA website under the following cumulative conditions: Links can only be made to webpages that provide a link to the Legal Notice page. | |
Record name | 2,4-DIMETHYLPENTA-1,3-DIENE | |
Source | FDA Global Substance Registration System (GSRS) | |
URL | https://gsrs.ncats.nih.gov/ginas/app/beta/substances/6CJ16Y4NXU | |
Description | The FDA Global Substance Registration System (GSRS) enables the efficient and accurate exchange of information on what substances are in regulated products. Instead of relying on names, which vary across regulatory domains, countries, and regions, the GSRS knowledge base makes it possible for substances to be defined by standardized, scientific descriptions. | |
Explanation | Unless otherwise noted, the contents of the FDA website (www.fda.gov), both text and graphics, are not copyrighted. They are in the public domain and may be republished, reprinted and otherwise used freely by anyone without the need to obtain permission from FDA. Credit to the U.S. Food and Drug Administration as the source is appreciated but not required. | |
Retrosynthesis Analysis
AI-Powered Synthesis Planning: Our tool employs the Template_relevance Pistachio, Template_relevance Bkms_metabolic, Template_relevance Pistachio_ringbreaker, Template_relevance Reaxys, Template_relevance Reaxys_biocatalysis model, leveraging a vast database of chemical reactions to predict feasible synthetic routes.
One-Step Synthesis Focus: Specifically designed for one-step synthesis, it provides concise and direct routes for your target compounds, streamlining the synthesis process.
Accurate Predictions: Utilizing the extensive PISTACHIO, BKMS_METABOLIC, PISTACHIO_RINGBREAKER, REAXYS, REAXYS_BIOCATALYSIS database, our tool offers high-accuracy predictions, reflecting the latest in chemical research and data.
Strategy Settings
Precursor scoring | Relevance Heuristic |
---|---|
Min. plausibility | 0.01 |
Model | Template_relevance |
Template Set | Pistachio/Bkms_metabolic/Pistachio_ringbreaker/Reaxys/Reaxys_biocatalysis |
Top-N result to add to graph | 6 |
Feasible Synthetic Routes
Disclaimer and Information on In-Vitro Research Products
Please be aware that all articles and product information presented on BenchChem are intended solely for informational purposes. The products available for purchase on BenchChem are specifically designed for in-vitro studies, which are conducted outside of living organisms. In-vitro studies, derived from the Latin term "in glass," involve experiments performed in controlled laboratory settings using cells or tissues. It is important to note that these products are not categorized as medicines or drugs, and they have not received approval from the FDA for the prevention, treatment, or cure of any medical condition, ailment, or disease. We must emphasize that any form of bodily introduction of these products into humans or animals is strictly prohibited by law. It is essential to adhere to these guidelines to ensure compliance with legal and ethical standards in research and experimentation.