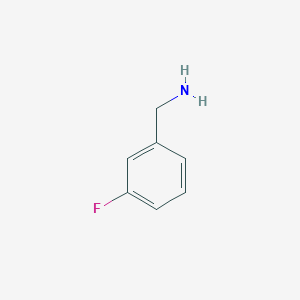
3-Fluorobenzylamine
Overview
Description
3-Fluorobenzylamine, also known as (3-Fluorophenyl)methanamine, is an organic compound with the molecular formula C7H8FN. It is a clear, colorless liquid that is primarily used in various chemical reactions and synthesis processes. The compound is characterized by the presence of a fluorine atom attached to the benzylamine structure, which imparts unique chemical properties .
Mechanism of Action
Target of Action
3-Fluorobenzylamine is a type of phenylmethylamine . Phenylmethylamines are compounds containing a phenylmethylamine moiety, which consists of a phenyl group substituted by a methanamine . .
Mode of Action
It is known that the compound has been used to study the rate of reaction of benzylamines with 1-chloro-2,4-dinitrobenzene and toluene-p-sulphonyl chloride . This suggests that this compound may interact with these compounds in its mode of action.
Biochemical Pathways
It has been used in the synthesis of substituted amino-sulfonamide protease inhibitors (pis) dpc 681 and dpc 684 . This suggests that this compound may play a role in the biochemical pathways related to these protease inhibitors.
Result of Action
Given its use in the synthesis of substituted amino-sulfonamide protease inhibitors (pis) dpc 681 and dpc 684 , it can be inferred that the compound may have an effect on the activity of certain proteases.
Action Environment
It is known that the compound is sensitive to air , suggesting that its action and stability may be influenced by exposure to oxygen.
Preparation Methods
Synthetic Routes and Reaction Conditions: 3-Fluorobenzylamine can be synthesized through several methods. One common approach involves the reaction of 3-fluorobenzyl chloride with ammonia or an amine under controlled conditions. Another method includes the reduction of 3-fluoronitrobenzene using hydrogen in the presence of a catalyst .
Industrial Production Methods: In industrial settings, this compound is often produced through large-scale chemical reactions involving the aforementioned methods. The process typically requires precise control of temperature, pressure, and the use of catalysts to ensure high yield and purity .
Chemical Reactions Analysis
Types of Reactions: 3-Fluorobenzylamine undergoes various chemical reactions, including:
Oxidation: It can be oxidized to form corresponding aldehydes or acids.
Reduction: The compound can be reduced to form different amines.
Substitution: It participates in nucleophilic substitution reactions, where the fluorine atom can be replaced by other substituents.
Common Reagents and Conditions:
Oxidation: Common oxidizing agents include potassium permanganate and chromium trioxide.
Reduction: Hydrogen gas in the presence of palladium or platinum catalysts is often used.
Substitution: Reagents like sodium hydride or lithium aluminum hydride are commonly employed.
Major Products:
Oxidation: 3-Fluorobenzaldehyde, 3-Fluorobenzoic acid.
Reduction: Various substituted amines.
Substitution: Compounds with different substituents replacing the fluorine atom.
Scientific Research Applications
3-Fluorobenzylamine has a wide range of applications in scientific research:
Chemistry: It is used as a building block in the synthesis of various organic compounds, including pharmaceuticals and agrochemicals.
Biology: The compound is utilized in the study of enzyme reactions and as a precursor in the synthesis of enzyme inhibitors.
Medicine: It plays a role in the development of drugs, particularly in the synthesis of protease inhibitors used in antiviral therapies.
Industry: this compound is employed in the production of specialty chemicals and materials
Comparison with Similar Compounds
- 4-Fluorobenzylamine
- 3-Chlorobenzylamine
- 4-Methoxybenzylamine
- 2,6-Difluorobenzylamine
Comparison: 3-Fluorobenzylamine is unique due to the position of the fluorine atom on the benzylamine structure, which significantly influences its chemical reactivity and biological activity. Compared to 4-Fluorobenzylamine, the 3-position fluorine atom in this compound offers different steric and electronic effects, making it suitable for specific applications in chemical synthesis and drug development .
Biological Activity
3-Fluorobenzylamine (3F-BnNH₂) is a compound of significant interest in medicinal chemistry due to its diverse biological activities. This article explores its pharmacological properties, mechanism of action, and potential therapeutic applications, supported by relevant case studies and research findings.
This compound is characterized by its molecular formula and a molecular weight of approximately 141.14 g/mol. Its structure features a fluorine atom positioned on the benzyl group, which influences its biological activity and interaction with various biological targets.
The biological activity of this compound can be attributed to its ability to act as a ligand for various receptors and enzymes. Notably, it has been shown to interact with the SARS-CoV papain-like protease (PLpro), which is crucial for viral replication. Research indicates that derivatives of this compound exhibit potent inhibitory effects against PLpro, with IC50 values significantly lower than those of non-fluorinated analogues. For instance, one study reported an IC50 value of 0.15 ± 0.01 µM for a compound related to 3F-BnNH₂, underscoring its potential as an antiviral agent against coronaviruses .
Antiviral Activity
Research has demonstrated that this compound and its derivatives possess substantial antiviral properties. A study highlighted that compounds featuring the 3-fluorobenzyl moiety exhibited effective inhibition against the replication of enterovirus EV71, with EC50 values ranging from 0.3 to 1.4 µM . These findings suggest that modifications in the benzyl group can enhance selectivity and potency against viral targets.
Cytotoxicity
While exploring the cytotoxic effects of this compound, it was noted that certain derivatives showed promising results against various cancer cell lines. However, some exhibited high cytotoxicity alongside antiviral activity, indicating a need for careful evaluation in therapeutic contexts. For example, the cytotoxicity was assessed using a reduction assay on rhabdomyosarcoma cells, revealing a complex relationship between antiviral efficacy and cellular toxicity .
Comparative Analysis of Biological Activity
The following table summarizes key findings related to the biological activity of this compound and its derivatives:
Compound | Target | IC50/EC50 Value | Notes |
---|---|---|---|
This compound | SARS-CoV PLpro | 0.15 ± 0.01 µM | High binding affinity |
Derivative 1 | EV71 | EC50: 0.3 - 1.4 µM | Effective antiviral activity |
Derivative 2 | Cancer Cell Lines | CC50: variable | High cytotoxicity observed |
Case Studies
- Inhibition of SARS-CoV PLpro : A detailed structural analysis revealed that specific substitutions on the benzyl group significantly enhanced binding affinity to PLpro, highlighting the potential for developing broad-spectrum antiviral drugs .
- Antiviral Efficacy Against EV71 : In vitro studies demonstrated that several analogues of this compound effectively reduced cytopathic effects in infected cells while maintaining lower toxicity levels compared to parent compounds .
Properties
IUPAC Name |
(3-fluorophenyl)methanamine | |
---|---|---|
Source | PubChem | |
URL | https://pubchem.ncbi.nlm.nih.gov | |
Description | Data deposited in or computed by PubChem | |
InChI |
InChI=1S/C7H8FN/c8-7-3-1-2-6(4-7)5-9/h1-4H,5,9H2 | |
Source | PubChem | |
URL | https://pubchem.ncbi.nlm.nih.gov | |
Description | Data deposited in or computed by PubChem | |
InChI Key |
QVSVMNXRLWSNGS-UHFFFAOYSA-N | |
Source | PubChem | |
URL | https://pubchem.ncbi.nlm.nih.gov | |
Description | Data deposited in or computed by PubChem | |
Canonical SMILES |
C1=CC(=CC(=C1)F)CN | |
Source | PubChem | |
URL | https://pubchem.ncbi.nlm.nih.gov | |
Description | Data deposited in or computed by PubChem | |
Molecular Formula |
C7H8FN | |
Source | PubChem | |
URL | https://pubchem.ncbi.nlm.nih.gov | |
Description | Data deposited in or computed by PubChem | |
DSSTOX Substance ID |
DTXSID8059219 | |
Record name | Benzenemethanamine, 3-fluoro- | |
Source | EPA DSSTox | |
URL | https://comptox.epa.gov/dashboard/DTXSID8059219 | |
Description | DSSTox provides a high quality public chemistry resource for supporting improved predictive toxicology. | |
Molecular Weight |
125.14 g/mol | |
Source | PubChem | |
URL | https://pubchem.ncbi.nlm.nih.gov | |
Description | Data deposited in or computed by PubChem | |
CAS No. |
100-82-3 | |
Record name | 3-Fluorobenzylamine | |
Source | CAS Common Chemistry | |
URL | https://commonchemistry.cas.org/detail?cas_rn=100-82-3 | |
Description | CAS Common Chemistry is an open community resource for accessing chemical information. Nearly 500,000 chemical substances from CAS REGISTRY cover areas of community interest, including common and frequently regulated chemicals, and those relevant to high school and undergraduate chemistry classes. This chemical information, curated by our expert scientists, is provided in alignment with our mission as a division of the American Chemical Society. | |
Explanation | The data from CAS Common Chemistry is provided under a CC-BY-NC 4.0 license, unless otherwise stated. | |
Record name | Benzenemethanamine, 3-fluoro- | |
Source | ChemIDplus | |
URL | https://pubchem.ncbi.nlm.nih.gov/substance/?source=chemidplus&sourceid=0000100823 | |
Description | ChemIDplus is a free, web search system that provides access to the structure and nomenclature authority files used for the identification of chemical substances cited in National Library of Medicine (NLM) databases, including the TOXNET system. | |
Record name | Benzenemethanamine, 3-fluoro- | |
Source | EPA Chemicals under the TSCA | |
URL | https://www.epa.gov/chemicals-under-tsca | |
Description | EPA Chemicals under the Toxic Substances Control Act (TSCA) collection contains information on chemicals and their regulations under TSCA, including non-confidential content from the TSCA Chemical Substance Inventory and Chemical Data Reporting. | |
Record name | Benzenemethanamine, 3-fluoro- | |
Source | EPA DSSTox | |
URL | https://comptox.epa.gov/dashboard/DTXSID8059219 | |
Description | DSSTox provides a high quality public chemistry resource for supporting improved predictive toxicology. | |
Record name | 3-fluorobenzylamine | |
Source | European Chemicals Agency (ECHA) | |
URL | https://echa.europa.eu/substance-information/-/substanceinfo/100.002.629 | |
Description | The European Chemicals Agency (ECHA) is an agency of the European Union which is the driving force among regulatory authorities in implementing the EU's groundbreaking chemicals legislation for the benefit of human health and the environment as well as for innovation and competitiveness. | |
Explanation | Use of the information, documents and data from the ECHA website is subject to the terms and conditions of this Legal Notice, and subject to other binding limitations provided for under applicable law, the information, documents and data made available on the ECHA website may be reproduced, distributed and/or used, totally or in part, for non-commercial purposes provided that ECHA is acknowledged as the source: "Source: European Chemicals Agency, http://echa.europa.eu/". Such acknowledgement must be included in each copy of the material. ECHA permits and encourages organisations and individuals to create links to the ECHA website under the following cumulative conditions: Links can only be made to webpages that provide a link to the Legal Notice page. | |
Record name | 3-Fluorobenzylamine | |
Source | FDA Global Substance Registration System (GSRS) | |
URL | https://gsrs.ncats.nih.gov/ginas/app/beta/substances/6MGA6423TQ | |
Description | The FDA Global Substance Registration System (GSRS) enables the efficient and accurate exchange of information on what substances are in regulated products. Instead of relying on names, which vary across regulatory domains, countries, and regions, the GSRS knowledge base makes it possible for substances to be defined by standardized, scientific descriptions. | |
Explanation | Unless otherwise noted, the contents of the FDA website (www.fda.gov), both text and graphics, are not copyrighted. They are in the public domain and may be republished, reprinted and otherwise used freely by anyone without the need to obtain permission from FDA. Credit to the U.S. Food and Drug Administration as the source is appreciated but not required. | |
Retrosynthesis Analysis
AI-Powered Synthesis Planning: Our tool employs the Template_relevance Pistachio, Template_relevance Bkms_metabolic, Template_relevance Pistachio_ringbreaker, Template_relevance Reaxys, Template_relevance Reaxys_biocatalysis model, leveraging a vast database of chemical reactions to predict feasible synthetic routes.
One-Step Synthesis Focus: Specifically designed for one-step synthesis, it provides concise and direct routes for your target compounds, streamlining the synthesis process.
Accurate Predictions: Utilizing the extensive PISTACHIO, BKMS_METABOLIC, PISTACHIO_RINGBREAKER, REAXYS, REAXYS_BIOCATALYSIS database, our tool offers high-accuracy predictions, reflecting the latest in chemical research and data.
Strategy Settings
Precursor scoring | Relevance Heuristic |
---|---|
Min. plausibility | 0.01 |
Model | Template_relevance |
Template Set | Pistachio/Bkms_metabolic/Pistachio_ringbreaker/Reaxys/Reaxys_biocatalysis |
Top-N result to add to graph | 6 |
Feasible Synthetic Routes
Q1: What makes 3-fluorobenzylamine a molecule of interest in the fight against tuberculosis?
A: this compound, often found as a component of larger molecules, has shown promising in vitro activity against Mycobacterium tuberculosis. Specifically, N-butyl-3-fluorobenzylamine demonstrated significant tuberculostatic activity with a minimum inhibitory concentration (MIC) of 30 µg/mL. [, ] This suggests that incorporating this compound into the structure of new drugs could lead to the development of novel antituberculosis agents.
Q2: How does the structure of N-alkylbenzylamines, particularly the presence of 3-fluoro substituent, influence their activity against Mycobacterium tuberculosis?
A: Research indicates that the length of the N-alkyl chain and the presence of specific substituents on the benzene ring significantly impact the antimycobacterial activity of N-alkylbenzylamines. [, ] N-butyl-3-fluorobenzylamine emerged as the most potent compound within a series of tested derivatives. [] Interestingly, substituting the benzyl methylene group (CH2) or linking molecules through this group resulted in a complete loss of activity. [] These findings highlight the importance of both the N-alkyl chain length and the presence of specific substituents, like the 3-fluoro group, for optimal antimycobacterial activity.
Q3: Beyond its antimycobacterial activity, what other applications have been explored for this compound?
A: this compound has demonstrated potential in targeting the 26S proteasome, a crucial cellular machinery responsible for protein degradation. [] Researchers identified a peptoid, KDT-11, containing a terminal N-methyl-N-(this compound) moiety, which exhibited selective toxicity towards the Rpn13 subunit of the proteasome. [] This selectivity makes it a promising lead for developing new therapeutics targeting specific proteasome subunits, particularly in the context of cancer, where Rpn13 is often overexpressed. []
Q4: What are the limitations of current research on this compound and future directions for exploration?
A: The current research on this compound, while promising, is primarily based on in vitro studies. Further investigations are needed to evaluate its in vivo efficacy, pharmacokinetic properties, and potential toxicity. [, , ] Exploring different drug delivery systems and optimizing the structure of this compound-containing compounds could enhance its bioavailability and therapeutic potential. [] Additionally, understanding the precise mechanism of action of this compound against Mycobacterium tuberculosis and the 26S proteasome will be crucial for rational drug design and development.
Disclaimer and Information on In-Vitro Research Products
Please be aware that all articles and product information presented on BenchChem are intended solely for informational purposes. The products available for purchase on BenchChem are specifically designed for in-vitro studies, which are conducted outside of living organisms. In-vitro studies, derived from the Latin term "in glass," involve experiments performed in controlled laboratory settings using cells or tissues. It is important to note that these products are not categorized as medicines or drugs, and they have not received approval from the FDA for the prevention, treatment, or cure of any medical condition, ailment, or disease. We must emphasize that any form of bodily introduction of these products into humans or animals is strictly prohibited by law. It is essential to adhere to these guidelines to ensure compliance with legal and ethical standards in research and experimentation.