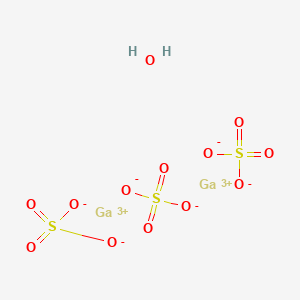
Gallium(III) sulfate hydrate
Overview
Description
Gallium(III) sulfate hydrate is a chemical compound with the formula Ga₂(SO₄)₃·xH₂O. It is a white solid that is slightly soluble in water and sulfuric acid. This compound is known for its use in the preparation of other gallium compounds and its role as a catalyst in various chemical reactions .
Mechanism of Action
Target of Action
Gallium(III) sulfate hydrate primarily targets the essential transcription enzyme RNA polymerase . This enzyme plays a crucial role in the synthesis of RNA from a DNA template, a process vital for gene expression.
Mode of Action
The compound interacts with its target, the RNA polymerase, to suppress RNA synthesis . This interaction results in reduced metabolic rates and energy utilization within the cell .
Pharmacokinetics
It’s known that gallium metal dissolves in sulfuric acid to form solutions containing [ga(oh2)6]3+ and so42− ions . This suggests that the compound may be soluble in certain biological environments, potentially influencing its bioavailability.
Result of Action
The primary molecular effect of this compound’s action is the suppression of RNA synthesis . This leads to reduced metabolic rates and energy utilization at the cellular level . The compound’s action can also result in decreased protein production due to the role of RNA in protein synthesis.
Action Environment
The action of this compound can be influenced by environmental factors. For instance, the formation of a gallium(III) sulfato complex is favored with increasing temperature . This suggests that the compound’s action, efficacy, and stability may vary depending on the temperature of its environment. Additionally, the compound is known to be slightly soluble in water , which could also influence its action in aqueous biological environments.
Biochemical Analysis
Molecular Mechanism
Gallium compounds are known to bind to biomolecules that normally bind iron, potentially inhibiting enzymes and disrupting gene expression .
Temporal Effects in Laboratory Settings
Gallium compounds are generally stable and do not degrade significantly over time .
Dosage Effects in Animal Models
Gallium compounds have been shown to have therapeutic effects at low doses and toxic effects at high doses in some animal models .
Metabolic Pathways
Gallium compounds are known to interfere with iron metabolism, potentially affecting metabolic flux and metabolite levels .
Transport and Distribution
Gallium compounds are known to be taken up by cells via mechanisms normally used for iron uptake .
Subcellular Localization
Gallium compounds are known to accumulate in lysosomes and other subcellular compartments .
Preparation Methods
Synthetic Routes and Reaction Conditions: Gallium(III) sulfate hydrate can be synthesized by dissolving gallium metal in sulfuric acid, resulting in a solution containing [Ga(OH₂)₆]³⁺ and SO₄²⁻ ions. The octadecahydrate form, Ga₂(SO₄)₃·18H₂O, crystallizes from these solutions at room temperature . The reaction can be represented as: [ 2 \text{Ga} + 3 \text{H}_2\text{SO}_4 \rightarrow \text{Ga}_2(\text{SO}_4)_3 + 3 \text{H}_2 ]
Industrial Production Methods: In industrial settings, this compound is often produced by reacting hydroxygallium diacetate with sulfuric acid at 90°C for two days, followed by drying in a vacuum to obtain the anhydrous form . The reaction is as follows: [ 2 \text{Ga(CH}_3\text{COO)}_2\text{OH} + 3 \text{H}_2\text{SO}_4 \rightarrow \text{Ga}_2(\text{SO}_4)_3 + 4 \text{CH}_3\text{COOH} + 2 \text{H}_2\text{O} ]
Types of Reactions:
-
Oxidation: When heated above 680°C, gallium(III) sulfate decomposes to form gallium(III) oxide and sulfur trioxide . [ \text{Ga}_2(\text{SO}_4)_3 \rightarrow \text{Ga}_2\text{O}_3 + 3 \text{SO}_3 ]
-
Precipitation: A solution of gallium(III) sulfate in water mixed with zinc sulfate can precipitate zinc gallate . [ \text{Ga}_2(\text{SO}_4)_3 + 3 \text{ZnSO}_4 \rightarrow 2 \text{ZnGa}_2\text{O}_4 + 3 \text{SO}_3 ]
Common Reagents and Conditions:
Sulfuric Acid: Used in the synthesis and dissolution processes.
Zinc Sulfate: Used in precipitation reactions to form zinc gallate.
Major Products:
Gallium(III) Oxide: Formed upon decomposition.
Zinc Gallate: Formed in precipitation reactions.
Scientific Research Applications
Gallium(III) sulfate hydrate has several applications in scientific research:
Material Science: Used in the preparation of gallium oxide, which is important in semiconductor technology.
Chemical Synthesis: Employed in the synthesis of various gallium compounds.
Comparison with Similar Compounds
Aluminium Sulfate: Similar in structure and used in water treatment.
Indium(III) Sulfate: Another group 13 metal sulfate with similar properties.
Uniqueness: Gallium(III) sulfate hydrate is unique due to its specific catalytic properties and its role in the preparation of high-purity gallium compounds. Its ability to form stable hydrates and its use in semiconductor technology further distinguish it from similar compounds .
Properties
IUPAC Name |
digallium;trisulfate;hydrate | |
---|---|---|
Source | PubChem | |
URL | https://pubchem.ncbi.nlm.nih.gov | |
Description | Data deposited in or computed by PubChem | |
InChI |
InChI=1S/2Ga.3H2O4S.H2O/c;;3*1-5(2,3)4;/h;;3*(H2,1,2,3,4);1H2/q2*+3;;;;/p-6 | |
Source | PubChem | |
URL | https://pubchem.ncbi.nlm.nih.gov | |
Description | Data deposited in or computed by PubChem | |
InChI Key |
CMNGAUGWXGMLDK-UHFFFAOYSA-H | |
Source | PubChem | |
URL | https://pubchem.ncbi.nlm.nih.gov | |
Description | Data deposited in or computed by PubChem | |
Canonical SMILES |
O.[O-]S(=O)(=O)[O-].[O-]S(=O)(=O)[O-].[O-]S(=O)(=O)[O-].[Ga+3].[Ga+3] | |
Source | PubChem | |
URL | https://pubchem.ncbi.nlm.nih.gov | |
Description | Data deposited in or computed by PubChem | |
Molecular Formula |
Ga2H2O13S3 | |
Source | PubChem | |
URL | https://pubchem.ncbi.nlm.nih.gov | |
Description | Data deposited in or computed by PubChem | |
Molecular Weight |
445.7 g/mol | |
Source | PubChem | |
URL | https://pubchem.ncbi.nlm.nih.gov | |
Description | Data deposited in or computed by PubChem | |
Synthesis routes and methods
Procedure details
Retrosynthesis Analysis
AI-Powered Synthesis Planning: Our tool employs the Template_relevance Pistachio, Template_relevance Bkms_metabolic, Template_relevance Pistachio_ringbreaker, Template_relevance Reaxys, Template_relevance Reaxys_biocatalysis model, leveraging a vast database of chemical reactions to predict feasible synthetic routes.
One-Step Synthesis Focus: Specifically designed for one-step synthesis, it provides concise and direct routes for your target compounds, streamlining the synthesis process.
Accurate Predictions: Utilizing the extensive PISTACHIO, BKMS_METABOLIC, PISTACHIO_RINGBREAKER, REAXYS, REAXYS_BIOCATALYSIS database, our tool offers high-accuracy predictions, reflecting the latest in chemical research and data.
Strategy Settings
Precursor scoring | Relevance Heuristic |
---|---|
Min. plausibility | 0.01 |
Model | Template_relevance |
Template Set | Pistachio/Bkms_metabolic/Pistachio_ringbreaker/Reaxys/Reaxys_biocatalysis |
Top-N result to add to graph | 6 |
Feasible Synthetic Routes
Disclaimer and Information on In-Vitro Research Products
Please be aware that all articles and product information presented on BenchChem are intended solely for informational purposes. The products available for purchase on BenchChem are specifically designed for in-vitro studies, which are conducted outside of living organisms. In-vitro studies, derived from the Latin term "in glass," involve experiments performed in controlled laboratory settings using cells or tissues. It is important to note that these products are not categorized as medicines or drugs, and they have not received approval from the FDA for the prevention, treatment, or cure of any medical condition, ailment, or disease. We must emphasize that any form of bodily introduction of these products into humans or animals is strictly prohibited by law. It is essential to adhere to these guidelines to ensure compliance with legal and ethical standards in research and experimentation.