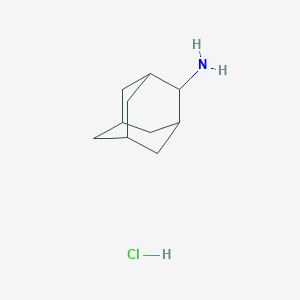
2-Aminoadamantane hydrochloride
Overview
Description
The HPLC assay of 2-adamantylamine hydrochloride after pre-column derivatization with 4-fluoro-7-nitro-2,1,3-benzoxadiazole has been studied.
Mechanism of Action
Target of Action
It’s known that adamantane derivatives have been found to interact with various targets, such as the camphor 5-monooxygenase in pseudomonas putida .
Mode of Action
It’s known that adamantane derivatives can interact with their targets in a variety of ways, often leading to changes in the target’s function .
Biochemical Pathways
Adamantane derivatives are known for their high reactivity, which offers extensive opportunities for their utilization as starting materials for the synthesis of various functional adamantane derivatives .
Pharmacokinetics
The adamantane moiety is widely applied in the design and synthesis of new drug delivery systems due to its lipophilicity and ability to ensure drug stability, resulting in enhanced pharmacokinetics of the modified drug candidates .
Result of Action
It’s known that adamantane derivatives have been used in the synthesis of various functional adamantane derivatives, monomers, thermally stable and high-energy fuels and oils, bioactive compounds, pharmaceuticals, and higher diamond-like bulky polymers such as diamondoids .
Properties
IUPAC Name |
adamantan-2-amine;hydrochloride | |
---|---|---|
Source | PubChem | |
URL | https://pubchem.ncbi.nlm.nih.gov | |
Description | Data deposited in or computed by PubChem | |
InChI |
InChI=1S/C10H17N.ClH/c11-10-8-2-6-1-7(4-8)5-9(10)3-6;/h6-10H,1-5,11H2;1H | |
Source | PubChem | |
URL | https://pubchem.ncbi.nlm.nih.gov | |
Description | Data deposited in or computed by PubChem | |
InChI Key |
WLDWDRZITJEWRJ-UHFFFAOYSA-N | |
Source | PubChem | |
URL | https://pubchem.ncbi.nlm.nih.gov | |
Description | Data deposited in or computed by PubChem | |
Canonical SMILES |
C1C2CC3CC1CC(C2)C3N.Cl | |
Source | PubChem | |
URL | https://pubchem.ncbi.nlm.nih.gov | |
Description | Data deposited in or computed by PubChem | |
Molecular Formula |
C10H18ClN | |
Source | PubChem | |
URL | https://pubchem.ncbi.nlm.nih.gov | |
Description | Data deposited in or computed by PubChem | |
Related CAS |
13074-39-0 (Parent) | |
Record name | 2-Adamantanamine, hydrochloride | |
Source | ChemIDplus | |
URL | https://pubchem.ncbi.nlm.nih.gov/substance/?source=chemidplus&sourceid=0010523689 | |
Description | ChemIDplus is a free, web search system that provides access to the structure and nomenclature authority files used for the identification of chemical substances cited in National Library of Medicine (NLM) databases, including the TOXNET system. | |
DSSTOX Substance ID |
DTXSID60147021 | |
Record name | 2-Adamantanamine, hydrochloride | |
Source | EPA DSSTox | |
URL | https://comptox.epa.gov/dashboard/DTXSID60147021 | |
Description | DSSTox provides a high quality public chemistry resource for supporting improved predictive toxicology. | |
Molecular Weight |
187.71 g/mol | |
Source | PubChem | |
URL | https://pubchem.ncbi.nlm.nih.gov | |
Description | Data deposited in or computed by PubChem | |
CAS No. |
10523-68-9 | |
Record name | 2-Adamantanamine, hydrochloride | |
Source | CAS Common Chemistry | |
URL | https://commonchemistry.cas.org/detail?cas_rn=10523-68-9 | |
Description | CAS Common Chemistry is an open community resource for accessing chemical information. Nearly 500,000 chemical substances from CAS REGISTRY cover areas of community interest, including common and frequently regulated chemicals, and those relevant to high school and undergraduate chemistry classes. This chemical information, curated by our expert scientists, is provided in alignment with our mission as a division of the American Chemical Society. | |
Explanation | The data from CAS Common Chemistry is provided under a CC-BY-NC 4.0 license, unless otherwise stated. | |
Record name | 2-Adamantanamine, hydrochloride | |
Source | ChemIDplus | |
URL | https://pubchem.ncbi.nlm.nih.gov/substance/?source=chemidplus&sourceid=0010523689 | |
Description | ChemIDplus is a free, web search system that provides access to the structure and nomenclature authority files used for the identification of chemical substances cited in National Library of Medicine (NLM) databases, including the TOXNET system. | |
Record name | 2-Adamantanamine, hydrochloride | |
Source | EPA DSSTox | |
URL | https://comptox.epa.gov/dashboard/DTXSID60147021 | |
Description | DSSTox provides a high quality public chemistry resource for supporting improved predictive toxicology. | |
Record name | Tricyclo[3.3.1.13.7]dec-2-ylamine hydrochloride | |
Source | European Chemicals Agency (ECHA) | |
URL | https://echa.europa.eu/substance-information/-/substanceinfo/100.030.964 | |
Description | The European Chemicals Agency (ECHA) is an agency of the European Union which is the driving force among regulatory authorities in implementing the EU's groundbreaking chemicals legislation for the benefit of human health and the environment as well as for innovation and competitiveness. | |
Explanation | Use of the information, documents and data from the ECHA website is subject to the terms and conditions of this Legal Notice, and subject to other binding limitations provided for under applicable law, the information, documents and data made available on the ECHA website may be reproduced, distributed and/or used, totally or in part, for non-commercial purposes provided that ECHA is acknowledged as the source: "Source: European Chemicals Agency, http://echa.europa.eu/". Such acknowledgement must be included in each copy of the material. ECHA permits and encourages organisations and individuals to create links to the ECHA website under the following cumulative conditions: Links can only be made to webpages that provide a link to the Legal Notice page. | |
Retrosynthesis Analysis
AI-Powered Synthesis Planning: Our tool employs the Template_relevance Pistachio, Template_relevance Bkms_metabolic, Template_relevance Pistachio_ringbreaker, Template_relevance Reaxys, Template_relevance Reaxys_biocatalysis model, leveraging a vast database of chemical reactions to predict feasible synthetic routes.
One-Step Synthesis Focus: Specifically designed for one-step synthesis, it provides concise and direct routes for your target compounds, streamlining the synthesis process.
Accurate Predictions: Utilizing the extensive PISTACHIO, BKMS_METABOLIC, PISTACHIO_RINGBREAKER, REAXYS, REAXYS_BIOCATALYSIS database, our tool offers high-accuracy predictions, reflecting the latest in chemical research and data.
Strategy Settings
Precursor scoring | Relevance Heuristic |
---|---|
Min. plausibility | 0.01 |
Model | Template_relevance |
Template Set | Pistachio/Bkms_metabolic/Pistachio_ringbreaker/Reaxys/Reaxys_biocatalysis |
Top-N result to add to graph | 6 |
Feasible Synthetic Routes
Q1: What are the analytical methods commonly used to quantify 2-Adamantanamine hydrochloride in biological samples?
A1: Several analytical methods have been developed for the quantification of 2-Adamantanamine hydrochloride in biological samples. One approach utilizes high-performance liquid chromatography (HPLC) coupled with fluorescence detection. This method involves derivatizing 2-Adamantanamine hydrochloride with reagents like 4-fluoro-7-nitro-2,1,3-benzoxadiazole (NBD-F) or o-phthalaldehyde (OPA) and 1-thio-beta-D-glucose (TG) to enhance detectability. These methods have demonstrated good sensitivity and reproducibility for determining 2-Adamantanamine hydrochloride concentrations in human plasma [, ]. Another method uses HPLC coupled with tandem mass spectrometry (HPLC-MS/MS) []. This technique provides high selectivity and sensitivity for quantifying 2-Adamantanamine hydrochloride, even in complex matrices like whole blood.
Q2: Can you explain the structural features of 2-Adamantanamine hydrochloride and its molecular weight?
A2: 2-Adamantanamine hydrochloride, also known as 2-Aminoadamantane hydrochloride, is an organic compound with the molecular formula C10H18ClN. Its molecular weight is 187.71 g/mol. The structure consists of an adamantane cage, which is a highly stable, symmetrical, and three-dimensional hydrocarbon molecule. An amino group (-NH2) is attached to the adamantane cage at the 2-position. The hydrochloride salt form refers to the compound's association with a hydrochloric acid (HCl) molecule, resulting in the presence of a chloride ion (Cl-) in the structure.
Q3: What are the known applications of 2-Adamantanamine hydrochloride in scientific research?
A3: 2-Adamantanamine hydrochloride has found utility in various scientific research areas. In pharmacology, it serves as a valuable tool in binding studies to investigate the interaction of adamantane derivatives with proteins like human α1-acid glycoprotein []. Additionally, 2-Adamantanamine hydrochloride is employed as an internal standard in analytical chemistry for the quantification of other compounds with similar structures, such as coumarin–monoterpene conjugates, using techniques like HPLC-MS/MS [].
Q4: Are there any reported structure-activity relationship (SAR) studies involving 2-Adamantanamine hydrochloride or its derivatives?
A4: While specific SAR studies focusing on 2-Adamantanamine hydrochloride are limited in the provided research, investigations into related adamantane derivatives, like amantadine (1-adamantanamine hydrochloride) and its analogs, offer insights into the impact of structural modifications on biological activity. Research has demonstrated that alterations in the adamantane ring substituents can significantly influence binding affinity to targets like human α1-acid glycoprotein []. These findings suggest that exploring structural variations in 2-Adamantanamine hydrochloride could lead to the discovery of compounds with tailored properties and potential applications in diverse fields.
Disclaimer and Information on In-Vitro Research Products
Please be aware that all articles and product information presented on BenchChem are intended solely for informational purposes. The products available for purchase on BenchChem are specifically designed for in-vitro studies, which are conducted outside of living organisms. In-vitro studies, derived from the Latin term "in glass," involve experiments performed in controlled laboratory settings using cells or tissues. It is important to note that these products are not categorized as medicines or drugs, and they have not received approval from the FDA for the prevention, treatment, or cure of any medical condition, ailment, or disease. We must emphasize that any form of bodily introduction of these products into humans or animals is strictly prohibited by law. It is essential to adhere to these guidelines to ensure compliance with legal and ethical standards in research and experimentation.