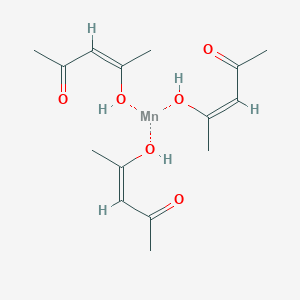
Manganese(III)acetylacetonate
Overview
Description
Manganese(III) acetylacetonate is a coordination complex with the chemical formula Mn(C₅H₇O₂)₃. It is a red-brown solid that is soluble in organic solvents. This compound is widely used in various fields due to its unique properties, such as its ability to act as an oxidizing agent and a catalyst in organic synthesis .
Mechanism of Action
Manganese(III) acetylacetonate, also known as Manganese(3+);(Z)-4-oxopent-2-en-2-olate, is a fascinating compound with a wide range of applications in the chemical industry . This article aims to provide a comprehensive overview of its mechanism of action, covering its primary targets, mode of action, biochemical pathways, pharmacokinetics, results of action, and the influence of environmental factors on its action.
Target of Action
Manganese(III) acetylacetonate primarily targets phenols, β-dicarbonyl compounds, and thiols, acting as a one-electron oxidizer . It also serves as a catalyst for the polymerization of alkenes and hardening of alkyd enamels .
Mode of Action
The compound interacts with its targets through oxidation, where it donates an electron to the target molecule . In certain reactions, it acts as a catalyst, accelerating the reaction rate without being consumed in the process .
Biochemical Pathways
Manganese(III) acetylacetonate is involved in various biochemical pathways. For instance, it plays a crucial role in the synthesis of manganese oxide cathode materials for lithium batteries . It also participates in the polymerization of alkenes . Furthermore, it’s used in the RAFT polymerization process, serving as a radical initiator .
Result of Action
The result of Manganese(III) acetylacetonate’s action is multifaceted. It can oxidize phenols, β-dicarbonyl compounds, and thiols . As a catalyst, it aids in the polymerization of alkenes and hardening of alkyd enamels . Moreover, it contributes to the synthesis of manganese oxide cathode materials for lithium batteries .
Action Environment
The action of Manganese(III) acetylacetonate is significantly influenced by environmental factors. For instance, the choice of solvent and temperature during synthesis affects the stability of the compound and the formation of its modifications . Low-polarity solvents with a low dielectric permittivity enhance intermolecular interactions, leading to the formation of specific modifications .
Biochemical Analysis
Cellular Effects
It is known to influence cell function, including impacts on cell signaling pathways, gene expression, and cellular metabolism
Molecular Mechanism
The molecular mechanism of Manganese(III)acetylacetonate involves its interactions at the molecular level, including binding interactions with biomolecules, enzyme inhibition or activation, and changes in gene expression
Temporal Effects in Laboratory Settings
In laboratory settings, the effects of this compound change over time. It has been observed that in the temperature range 140–240°C, this compound melts to form a different compound . At temperatures of 500–550°С, it decomposes to a mixture of different manganese oxides and carbon .
Preparation Methods
Synthetic Routes and Reaction Conditions: Manganese(III) acetylacetonate can be synthesized through several methods:
Reduction of Potassium Permanganate with Acetylacetone: This method involves the reduction of potassium permanganate (KMnO₄) with acetylacetone (C₅H₈O₂) in the presence of a neutralizing agent such as sodium acetate.
Co-proportionation of Manganese(II) and Potassium Permanganate: In this method, solutions of manganese(II) salts and potassium permanganate are mixed in the presence of acetylacetone.
Oxidation of Manganese(II) Acetylacetonate: Manganese(II) acetylacetonate can be oxidized to manganese(III) acetylacetonate using an oxidizing agent such as hydrogen peroxide (H₂O₂) or oxygen (O₂).
Industrial Production Methods: Industrial production of manganese(III) acetylacetonate typically involves the reduction of potassium permanganate with acetylacetone. The reaction is carried out in large-scale reactors with precise control over temperature and pH to ensure high yield and purity .
Types of Reactions:
Reduction: It can be reduced to manganese(II) acetylacetonate under certain conditions.
Substitution: The acetylacetonate ligands can be substituted with other ligands in the presence of suitable reagents.
Common Reagents and Conditions:
Oxidation: Common oxidizing agents include hydrogen peroxide and oxygen.
Reduction: Reducing agents such as sodium borohydride (NaBH₄) can be used.
Substitution: Ligand substitution reactions can be carried out using various ligands such as phosphines and amines.
Major Products:
Scientific Research Applications
Manganese(III) acetylacetonate has a wide range of applications in scientific research:
Comparison with Similar Compounds
- Chromium(III) acetylacetonate: Used as a catalyst in organic synthesis and as a precursor for chromium oxide nanoparticles .
- Iron(III) acetylacetonate: Used in the synthesis of iron oxide nanoparticles and as a catalyst in various organic reactions .
- Cobalt(III) acetylacetonate: Used as a catalyst in the polymerization of olefins and as a precursor for cobalt oxide nanoparticles .
Properties
CAS No. |
14284-89-0 |
---|---|
Molecular Formula |
C15H24MnO6 |
Molecular Weight |
355.28 g/mol |
IUPAC Name |
4-hydroxypent-3-en-2-one;manganese |
InChI |
InChI=1S/3C5H8O2.Mn/c3*1-4(6)3-5(2)7;/h3*3,6H,1-2H3; |
InChI Key |
RDMHXWZYVFGYSF-UHFFFAOYSA-N |
SMILES |
CC(=CC(=O)C)O.CC(=CC(=O)C)O.CC(=CC(=O)C)O.[Mn] |
Isomeric SMILES |
C/C(=C/C(=O)C)/[O-].C/C(=C/C(=O)C)/[O-].C/C(=C/C(=O)C)/[O-].[Mn+3] |
Canonical SMILES |
CC(=CC(=O)C)O.CC(=CC(=O)C)O.CC(=CC(=O)C)O.[Mn] |
14284-89-0 | |
Pictograms |
Irritant |
Related CAS |
17272-66-1 (Parent) |
Synonyms |
acetyl acetonate acetyl acetonate, chromium (III) salt acetyl acetonate, copper (+2) salt acetyl acetonate, manganese (II) salt acetyl acetonate, manganese (III) salt acetyl acetonate, potassium salt acetyl acetonate, sodium salt acetylacetonate chromium(III) acetylacetonate copper acetylacetonate copper(II) acetylacetonate Cu(II) acetyl acetonate cupric acetylacetonate manganese acetylacetonate Nd(III)-acetylacetonate vanadyl acetylacetonate |
Origin of Product |
United States |
Retrosynthesis Analysis
AI-Powered Synthesis Planning: Our tool employs the Template_relevance Pistachio, Template_relevance Bkms_metabolic, Template_relevance Pistachio_ringbreaker, Template_relevance Reaxys, Template_relevance Reaxys_biocatalysis model, leveraging a vast database of chemical reactions to predict feasible synthetic routes.
One-Step Synthesis Focus: Specifically designed for one-step synthesis, it provides concise and direct routes for your target compounds, streamlining the synthesis process.
Accurate Predictions: Utilizing the extensive PISTACHIO, BKMS_METABOLIC, PISTACHIO_RINGBREAKER, REAXYS, REAXYS_BIOCATALYSIS database, our tool offers high-accuracy predictions, reflecting the latest in chemical research and data.
Strategy Settings
Precursor scoring | Relevance Heuristic |
---|---|
Min. plausibility | 0.01 |
Model | Template_relevance |
Template Set | Pistachio/Bkms_metabolic/Pistachio_ringbreaker/Reaxys/Reaxys_biocatalysis |
Top-N result to add to graph | 6 |
Feasible Synthetic Routes
Q1: What are the main catalytic applications of Mn(acac)3?
A1: Mn(acac)3 acts as a catalyst in various organic reactions, including:
- Oxidation reactions: Mn(acac)3 catalyzes the oxidation of phenols [, , ], alcohols [], sulfides [], and carbon-carbon double bonds []. It is particularly effective in reactions employing sodium chlorite as the oxidant [, , ].
- Polymerization reactions: Mn(acac)3 initiates the free-radical polymerization of monomers like methyl methacrylate, styrene, and acrylonitrile [, , , , ]. It exhibits monomer selectivity, with higher activity for acrylonitrile [].
- Phosphorylation reactions: Mn(acac)3 catalyzes the regioselective phosphorylation of tertiary enamides, primarily yielding β-phosphorylated products [].
Q2: How does the structure of Mn(acac)3 contribute to its catalytic activity?
A2: Mn(acac)3 possesses a labile acetylacetonate (acac) ligand. This lability facilitates ligand displacement reactions, generating reactive manganese species that can participate in various catalytic cycles [].
Q3: Can you provide an example of a reaction mechanism involving Mn(acac)3 as a catalyst?
A3: In the oxidation of sulfides to sulfoxides using sodium chlorite, Mn(acac)3 likely acts as an electron-transfer mediator. It facilitates the formation of a hypochlorite species from sodium chlorite, which then oxidizes the sulfide to the sulfoxide [].
Q4: How does Mn(acac)3 compare to other catalysts in terms of selectivity?
A4: Mn(acac)3 demonstrates unique selectivity in several reactions. For instance, in the phosphorylation of tertiary enamides, it favors β-phosphorylation, while other Lewis acid catalysts often lead to α-phosphorylated products [].
Q5: Are there any challenges associated with using Mn(acac)3 as a catalyst?
A5: One challenge is the potential for Mn(acac)3 to undergo decomposition during reactions, especially at elevated temperatures or prolonged reaction times []. This can limit its catalytic efficiency and require optimization of reaction conditions.
Q6: What is the stability of Mn(acac)3 under different conditions?
A6: Mn(acac)3 is sensitive to moisture and should be stored under inert conditions. It is generally stable at room temperature but can decompose at elevated temperatures [].
Q7: How does the presence of solvents or additives affect the stability and performance of Mn(acac)3?
A7: Solvents and additives can significantly impact the behavior of Mn(acac)3. For example:
- Dimethyl sulfoxide enhances the initiation efficiency of Mn(acac)3 in free-radical polymerization [].
- The choice of solvent can influence the regioselectivity of reactions catalyzed by Mn(acac)3. In the chlorination of alkyl phenyl ethers, using dichloromethane as the solvent favors para-selectivity [].
Q8: What is the molecular formula and weight of Mn(acac)3?
A8: The molecular formula of Mn(acac)3 is C15H21MnO6 and its molecular weight is 352.30 g/mol [].
Q9: What spectroscopic techniques are commonly used to characterize Mn(acac)3?
A9: Various spectroscopic techniques can be employed, including:
- Infrared (IR) spectroscopy: IR spectroscopy helps identify the presence of characteristic functional groups, such as the carbonyl groups in the acetylacetonate ligands [, , ].
- UV-Vis spectroscopy: UV-Vis spectroscopy can provide information about the electronic structure and coordination environment of the manganese(III) ion [, ].
- Electron paramagnetic resonance (EPR) spectroscopy: EPR spectroscopy is useful for studying the paramagnetic Mn(III) center and its interaction with the ligands [].
Q10: What are the applications of Mn(acac)3 in materials science?
A10: Mn(acac)3 serves as a precursor for synthesizing various manganese-containing materials:
- Manganese oxide nanoparticles: Mn(acac)3 can be used to prepare manganese oxide nanoparticles with potential applications in catalysis, energy storage, and biomedical fields [, ].
- Mn-doped zeolites: Mn(acac)3 acts as a source of manganese for incorporating Mn ions into zeolite frameworks, enhancing their catalytic properties in oxidation reactions [, ].
- Thin films: Mn(acac)3 is used in the preparation of manganese oxide thin films for applications in electrochromic devices and as electrode materials [, ].
Q11: What are the advantages of using Mn(acac)3 as a precursor in material synthesis?
A11: Mn(acac)3 offers several advantages, including:
Q12: Are there any efforts to develop greener synthetic routes for Mn(acac)3?
A13: Yes, researchers are exploring more environmentally benign methods for synthesizing Mn(acac)3, such as using water as a solvent or employing microwave irradiation to reduce reaction times and energy consumption [].
Disclaimer and Information on In-Vitro Research Products
Please be aware that all articles and product information presented on BenchChem are intended solely for informational purposes. The products available for purchase on BenchChem are specifically designed for in-vitro studies, which are conducted outside of living organisms. In-vitro studies, derived from the Latin term "in glass," involve experiments performed in controlled laboratory settings using cells or tissues. It is important to note that these products are not categorized as medicines or drugs, and they have not received approval from the FDA for the prevention, treatment, or cure of any medical condition, ailment, or disease. We must emphasize that any form of bodily introduction of these products into humans or animals is strictly prohibited by law. It is essential to adhere to these guidelines to ensure compliance with legal and ethical standards in research and experimentation.