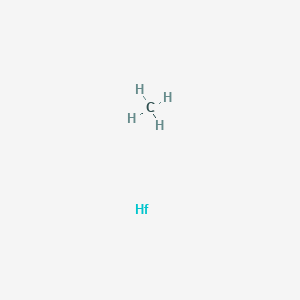
Hafnium carbide (HfC)
Overview
Description
Hafnium carbide is a chemical compound composed of hafnium and carbon. It is known for its exceptional properties, including a very high melting point of approximately 3,958 degrees Celsius, making it one of the most refractory materials known . Hafnium carbide is typically found as a black, odorless powder and has a cubic crystal structure .
Mechanism of Action
Target of Action
Hafnium Carbide (HfC) is primarily targeted for use in high-temperature applications due to its exceptional mechanical, thermal, and electrical properties . It is often used in the manufacture of protective coatings in industries such as nuclear power, aviation, and rocket technology .
Mode of Action
Hafnium Carbide (HfC) is usually carbon deficient, and its composition is often expressed as HfC x (x = 0.5 to 1.0). It has a cubic (rock-salt) crystal structure at any value of x . HfC powder is obtained by the reduction of hafnium (IV) oxide with carbon at 1,800 to 2,000 °C . High-purity HfC coatings can also be obtained by chemical vapor deposition from a gas mixture of methane, hydrogen, and vaporized hafnium (IV) chloride .
Biochemical Pathways
Its primary applications are in materials science and engineering, rather than biochemistry .
Pharmacokinetics
Its properties are more relevant in the context of materials science, where its high melting point, extreme hardness, and excellent thermal stability are of primary importance .
Result of Action
The result of HfC’s action is the formation of a material with a high melting point, extreme hardness, and excellent thermal stability . These properties make it suitable for use in high-temperature applications, such as protective coatings in various industries .
Action Environment
The action of HfC is influenced by environmental factors such as temperature and the presence of oxygen. For instance, HfC has a low oxidation resistance, with oxidation starting at temperatures as low as 430 °C . Additionally, the formation of HfC requires high temperatures (1,800 to 2,000 °C) and specific conditions (e.g., the presence of carbon and absence of oxygen) .
Preparation Methods
Synthetic Routes and Reaction Conditions: Hafnium carbide can be synthesized through several methods:
Carbothermic Reduction: This involves the reduction of hafnium(IV) oxide with carbon at temperatures ranging from 1,800 to 2,000 degrees Celsius. This method requires a long processing time to ensure the complete removal of oxygen.
Chemical Vapor Deposition (CVD): High-purity hafnium carbide coatings can be obtained by chemical vapor deposition from a gas mixture of methane, hydrogen, and vaporized hafnium(IV) chloride.
Sol-Gel Polycondensation: This method involves the use of sol-gel techniques to produce hafnium carbide powders.
Spark Plasma Sintering (SPS): This technique is used to produce dense hafnium carbide ceramics by applying a pulsed electric current to a powder compact.
Industrial Production Methods: Industrial production of hafnium carbide primarily relies on carbothermic reduction and chemical vapor deposition due to their efficiency in producing high-purity materials .
Types of Reactions:
Reduction: The compound can be reduced to its elemental form under specific conditions.
Common Reagents and Conditions:
Oxidation: Typically involves exposure to oxygen or air at elevated temperatures.
Reduction: Involves the use of reducing agents such as hydrogen or carbon at high temperatures.
Substitution: Requires the presence of reactive gases like nitrogen or ammonia at high temperatures.
Major Products Formed:
Oxidation: Forms hafnium dioxide (HfO2) and carbon dioxide (CO2).
Reduction: Produces elemental hafnium and carbon.
Substitution: Forms hafnium carbonitride (HfCN) and other similar compounds.
Scientific Research Applications
Hafnium carbide has a wide range of applications in scientific research due to its unique properties:
Chemistry: Used as a catalyst in various chemical reactions and as a material for high-temperature crucibles.
Biology and Medicine: While not commonly used directly in biological or medical applications, its high-temperature stability makes it useful in the development of medical devices that require sterilization at high temperatures.
Industry: Extensively used in the aerospace industry for components that must withstand extreme temperatures, such as rocket nozzles and leading edges of hypersonic vehicles.
Comparison with Similar Compounds
- Tantalum carbide (TaC)
- Zirconium carbide (ZrC)
- Niobium carbide (NbC)
- Hafnium carbonitride (HfCN)
Comparison:
- Melting Point: Hafnium carbide has one of the highest melting points among these compounds, making it particularly suitable for ultra-high-temperature applications .
- Hardness: Hafnium carbide is known for its extreme hardness, which is comparable to that of tantalum carbide and zirconium carbide .
- Oxidation Resistance: Hafnium carbide has lower oxidation resistance compared to some of its counterparts, such as zirconium carbide .
Hafnium carbide stands out due to its unique combination of high melting point, hardness, and thermal stability, making it a valuable material in various high-temperature and high-stress applications.
Properties
IUPAC Name |
hafnium;methane | |
---|---|---|
Source | PubChem | |
URL | https://pubchem.ncbi.nlm.nih.gov | |
Description | Data deposited in or computed by PubChem | |
InChI |
InChI=1S/CH4.Hf/h1H4; | |
Source | PubChem | |
URL | https://pubchem.ncbi.nlm.nih.gov | |
Description | Data deposited in or computed by PubChem | |
InChI Key |
WHJFNYXPKGDKBB-UHFFFAOYSA-N | |
Source | PubChem | |
URL | https://pubchem.ncbi.nlm.nih.gov | |
Description | Data deposited in or computed by PubChem | |
Canonical SMILES |
C.[Hf] | |
Source | PubChem | |
URL | https://pubchem.ncbi.nlm.nih.gov | |
Description | Data deposited in or computed by PubChem | |
Molecular Formula |
CH4Hf | |
Source | PubChem | |
URL | https://pubchem.ncbi.nlm.nih.gov | |
Description | Data deposited in or computed by PubChem | |
DSSTOX Substance ID |
DTXSID00923528 | |
Record name | Hafnium--methane (1/1) | |
Source | EPA DSSTox | |
URL | https://comptox.epa.gov/dashboard/DTXSID00923528 | |
Description | DSSTox provides a high quality public chemistry resource for supporting improved predictive toxicology. | |
Molecular Weight |
194.53 g/mol | |
Source | PubChem | |
URL | https://pubchem.ncbi.nlm.nih.gov | |
Description | Data deposited in or computed by PubChem | |
CAS No. |
12069-85-1 | |
Record name | Hafnium carbide (HfC) | |
Source | ChemIDplus | |
URL | https://pubchem.ncbi.nlm.nih.gov/substance/?source=chemidplus&sourceid=0012069851 | |
Description | ChemIDplus is a free, web search system that provides access to the structure and nomenclature authority files used for the identification of chemical substances cited in National Library of Medicine (NLM) databases, including the TOXNET system. | |
Record name | Hafnium carbide (HfC) | |
Source | EPA Chemicals under the TSCA | |
URL | https://www.epa.gov/chemicals-under-tsca | |
Description | EPA Chemicals under the Toxic Substances Control Act (TSCA) collection contains information on chemicals and their regulations under TSCA, including non-confidential content from the TSCA Chemical Substance Inventory and Chemical Data Reporting. | |
Record name | Hafnium--methane (1/1) | |
Source | EPA DSSTox | |
URL | https://comptox.epa.gov/dashboard/DTXSID00923528 | |
Description | DSSTox provides a high quality public chemistry resource for supporting improved predictive toxicology. | |
Retrosynthesis Analysis
AI-Powered Synthesis Planning: Our tool employs the Template_relevance Pistachio, Template_relevance Bkms_metabolic, Template_relevance Pistachio_ringbreaker, Template_relevance Reaxys, Template_relevance Reaxys_biocatalysis model, leveraging a vast database of chemical reactions to predict feasible synthetic routes.
One-Step Synthesis Focus: Specifically designed for one-step synthesis, it provides concise and direct routes for your target compounds, streamlining the synthesis process.
Accurate Predictions: Utilizing the extensive PISTACHIO, BKMS_METABOLIC, PISTACHIO_RINGBREAKER, REAXYS, REAXYS_BIOCATALYSIS database, our tool offers high-accuracy predictions, reflecting the latest in chemical research and data.
Strategy Settings
Precursor scoring | Relevance Heuristic |
---|---|
Min. plausibility | 0.01 |
Model | Template_relevance |
Template Set | Pistachio/Bkms_metabolic/Pistachio_ringbreaker/Reaxys/Reaxys_biocatalysis |
Top-N result to add to graph | 6 |
Feasible Synthetic Routes
Disclaimer and Information on In-Vitro Research Products
Please be aware that all articles and product information presented on BenchChem are intended solely for informational purposes. The products available for purchase on BenchChem are specifically designed for in-vitro studies, which are conducted outside of living organisms. In-vitro studies, derived from the Latin term "in glass," involve experiments performed in controlled laboratory settings using cells or tissues. It is important to note that these products are not categorized as medicines or drugs, and they have not received approval from the FDA for the prevention, treatment, or cure of any medical condition, ailment, or disease. We must emphasize that any form of bodily introduction of these products into humans or animals is strictly prohibited by law. It is essential to adhere to these guidelines to ensure compliance with legal and ethical standards in research and experimentation.