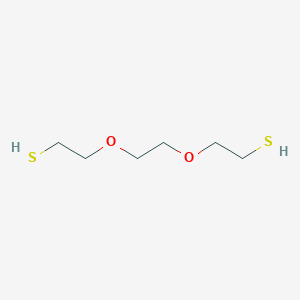
3,6-Dioxa-1,8-octanedithiol
Overview
Description
3,6-Dioxa-1,8-octanedithiol, also known as 3,6-Dioxa-1,8-octane-dithiol, is a dithiol compound with the molecular formula C6H14O2S2 and a molecular weight of 182.30 g/mol . This compound is characterized by the presence of two thiol groups (-SH) and two ether linkages (-O-), making it a versatile reagent in various chemical reactions and applications.
Mechanism of Action
Target of Action
The primary targets of 3,6-Dioxa-1,8-octanedithiol are dithiol compounds . It has been used as a model compound for the active sites of thioredoxins . Thioredoxins are proteins that play a crucial role in many biological processes, particularly in the formation of intramolecular disulfide bonds .
Mode of Action
this compound interacts with its targets through oxidation . A Pt(IV) complex has been used to study the oxidations of dithiol compounds by this compound . The oxidation products were characterized as ten- and six-membered ring compounds for this compound and dl-dithiothreitol (DTT), respectively, both containing an intramolecular disulfide bond .
Biochemical Pathways
The oxidation of dithiols by this compound affects the formation of intramolecular disulfide bonds . These bonds are very important in peptides and proteins and play crucial roles in many biological processes . The formation of these bonds has been of interest for the preparation of pharmaceuticals and fine chemicals .
Pharmacokinetics
It is known to be slightly soluble in water , which may impact its bioavailability.
Result of Action
The result of the action of this compound is the formation of cyclic disulfides . These cyclic disulfides are formed rapidly and quantitatively, making this compound an efficient reagent for this purpose .
Action Environment
The action of this compound can be influenced by environmental factors. For instance, the reaction conditions can affect the structure of poly (this compound) made by Reversible Radical Recombination Polymerization (R3P) using triethylamine (TEA), H2O2, and air .
Biochemical Analysis
Biochemical Properties
It has been used for cleaving peptides in the study to develop synthetic peptide serology to treat chronic chagas disease . It may also be used to develop non-malodorous scavenger in Fmoc-based peptide synthesis .
Cellular Effects
Its role in peptide cleavage suggests that it may have an impact on protein processing and turnover within cells .
Molecular Mechanism
The molecular mechanism of 3,6-Dioxa-1,8-octanedithiol involves its oxidation by a Pt(IV) complex . The oxidation products were characterized as ten- and six-membered ring compounds for this compound and dl-dithiothreitol (DTT), respectively, both containing an intramolecular disulfide bond .
Temporal Effects in Laboratory Settings
Its oxidation by a Pt(IV) complex was followed by stopped-flow spectrophotometry over a wide pH range .
Metabolic Pathways
Its role in peptide cleavage suggests that it may be involved in protein metabolism .
Preparation Methods
Synthetic Routes and Reaction Conditions
3,6-Dioxa-1,8-octanedithiol can be synthesized through the reaction of ethylene glycol with thiourea followed by hydrolysis. The reaction typically involves the following steps:
Formation of Ethylene Glycol Dithiocarbamate: Ethylene glycol reacts with thiourea in the presence of a base such as sodium hydroxide to form ethylene glycol dithiocarbamate.
Hydrolysis: The dithiocarbamate is then hydrolyzed using hydrochloric acid to yield this compound.
Industrial Production Methods
In industrial settings, the synthesis of this compound may involve optimized reaction conditions such as controlled temperature, pressure, and the use of catalysts to enhance yield and purity. The use of continuous flow reactors and advanced purification techniques can further improve the efficiency of production.
Chemical Reactions Analysis
Types of Reactions
3,6-Dioxa-1,8-octanedithiol undergoes various chemical reactions, including:
Oxidation: The thiol groups can be oxidized to form disulfides.
Reduction: The compound can be reduced to form the corresponding thiolates.
Substitution: The ether linkages can participate in nucleophilic substitution reactions.
Common Reagents and Conditions
Oxidation: Common oxidizing agents include hydrogen peroxide (H2O2) and iodine (I2).
Reduction: Reducing agents such as sodium borohydride (NaBH4) and lithium aluminum hydride (LiAlH4) are used.
Substitution: Nucleophiles such as alkoxides and amines can react with the ether linkages under basic conditions.
Major Products
Disulfides: Formed through oxidation of thiol groups.
Thiols: Formed through reduction of disulfides.
Substituted Ethers: Formed through nucleophilic substitution reactions.
Scientific Research Applications
3,6-Dioxa-1,8-octanedithiol has a wide range of applications in scientific research, including:
Comparison with Similar Compounds
Similar Compounds
1,2-Bis(2-chloroethoxy)ethane: Similar structure but with chloro groups instead of thiol groups.
1,2-Bis(2-iodoethoxy)ethane: Contains iodine atoms instead of thiol groups.
1,2-Bis(2-aminophenoxy)ethane: Features amino groups instead of thiol groups.
Uniqueness
3,6-Dioxa-1,8-octanedithiol is unique due to its dual thiol groups, which provide strong nucleophilic and reducing properties. This makes it particularly effective in forming disulfide bonds and participating in redox reactions, distinguishing it from other similar compounds .
Biological Activity
3,6-Dioxa-1,8-octanedithiol (DODT) is a compound of significant interest due to its unique chemical structure and diverse biological activities. This article explores its biological activity, including its role in organic synthesis, potential therapeutic applications, and interactions with biological systems.
Chemical Structure and Properties
This compound features a linear arrangement with two thiol groups (-SH) at either end of an octane chain, which contributes to its reactivity in various chemical reactions. The presence of these thiol groups allows DODT to participate in redox reactions and to act as a reducing agent in biochemical processes .
1. Role in Organic Synthesis
DODT serves as a versatile precursor in the synthesis of complex organic molecules. It has been utilized to synthesize various heterocyclic compounds, including bis(1,3,4-oxadiazoles) and bis(1,2,4-triazoles), demonstrating its utility in creating biologically relevant structures. The ability to functionalize the sulfur atoms in these derivatives further enhances their potential applications in medicinal chemistry.
2. Interactions with Thioredoxins
Research has shown that DODT can interact with thioredoxins, which are small redox proteins involved in cellular redox regulation. In a study exploring the oxidation of DODT by platinum(IV) anticancer drugs, it was found that DODT could be oxidized to form intramolecular disulfides. This reaction mimics the behavior of thioredoxin active sites and suggests that DODT may play a role in modulating redox states within cancer cells . The oxidation process involves multiple rate-determining steps that depend on the pH of the medium.
3. Alkylation Reactions
DODT has been employed as a scavenger during peptide cleavage processes. Its use has been associated with the formation of alkylation by-products when cleaving peptides containing methionine. Adjustments in cleavage conditions can mitigate these unwanted by-products, indicating that DODT's reactivity can be both beneficial and problematic depending on the context .
Case Study 1: Peptide Functionalization
In a study focused on peptide functionalization using DODT, researchers demonstrated its effectiveness in modifying polymeric materials for biomedical applications. The controlled functionalization allowed for enhanced interactions with biological systems, showcasing DODT's potential as a linker in drug delivery systems .
Case Study 2: Anticancer Applications
Another investigation highlighted DODT's role as a model compound for studying interactions with anticancer agents. The findings suggested that DODT could facilitate the delivery of platinum-based drugs to cancer cells by mimicking natural reductants present in biological systems . This property could be harnessed to improve the efficacy of existing chemotherapy treatments.
Summary of Findings
The biological activity of this compound is multifaceted:
Property | Description |
---|---|
Chemical Structure | Linear dithiol with two thiol groups |
Role in Synthesis | Precursor for heterocyclic compounds |
Redox Activity | Interacts with thioredoxins; oxidized to form disulfides |
Alkylation Reactions | Can form by-products during peptide cleavage |
Biomedical Applications | Potential use as a linker for drug delivery systems |
Properties
IUPAC Name |
2-[2-(2-sulfanylethoxy)ethoxy]ethanethiol | |
---|---|---|
Source | PubChem | |
URL | https://pubchem.ncbi.nlm.nih.gov | |
Description | Data deposited in or computed by PubChem | |
InChI |
InChI=1S/C6H14O2S2/c9-5-3-7-1-2-8-4-6-10/h9-10H,1-6H2 | |
Source | PubChem | |
URL | https://pubchem.ncbi.nlm.nih.gov | |
Description | Data deposited in or computed by PubChem | |
InChI Key |
HCZMHWVFVZAHCR-UHFFFAOYSA-N | |
Source | PubChem | |
URL | https://pubchem.ncbi.nlm.nih.gov | |
Description | Data deposited in or computed by PubChem | |
Canonical SMILES |
C(COCCS)OCCS | |
Source | PubChem | |
URL | https://pubchem.ncbi.nlm.nih.gov | |
Description | Data deposited in or computed by PubChem | |
Molecular Formula |
C6H14O2S2 | |
Source | PubChem | |
URL | https://pubchem.ncbi.nlm.nih.gov | |
Description | Data deposited in or computed by PubChem | |
DSSTOX Substance ID |
DTXSID9044588 | |
Record name | 2,2'-[Ethane-1,2-diylbis(oxy)]diethanethiol | |
Source | EPA DSSTox | |
URL | https://comptox.epa.gov/dashboard/DTXSID9044588 | |
Description | DSSTox provides a high quality public chemistry resource for supporting improved predictive toxicology. | |
Molecular Weight |
182.3 g/mol | |
Source | PubChem | |
URL | https://pubchem.ncbi.nlm.nih.gov | |
Description | Data deposited in or computed by PubChem | |
Physical Description |
Liquid | |
Record name | Ethanethiol, 2,2'-[1,2-ethanediylbis(oxy)]bis- | |
Source | EPA Chemicals under the TSCA | |
URL | https://www.epa.gov/chemicals-under-tsca | |
Description | EPA Chemicals under the Toxic Substances Control Act (TSCA) collection contains information on chemicals and their regulations under TSCA, including non-confidential content from the TSCA Chemical Substance Inventory and Chemical Data Reporting. | |
CAS No. |
14970-87-7, 68865-60-1 | |
Record name | 2,2′-(Ethylenedioxy)diethanethiol | |
Source | CAS Common Chemistry | |
URL | https://commonchemistry.cas.org/detail?cas_rn=14970-87-7 | |
Description | CAS Common Chemistry is an open community resource for accessing chemical information. Nearly 500,000 chemical substances from CAS REGISTRY cover areas of community interest, including common and frequently regulated chemicals, and those relevant to high school and undergraduate chemistry classes. This chemical information, curated by our expert scientists, is provided in alignment with our mission as a division of the American Chemical Society. | |
Explanation | The data from CAS Common Chemistry is provided under a CC-BY-NC 4.0 license, unless otherwise stated. | |
Record name | PEG dithiol | |
Source | CAS Common Chemistry | |
URL | https://commonchemistry.cas.org/detail?cas_rn=68865-60-1 | |
Description | CAS Common Chemistry is an open community resource for accessing chemical information. Nearly 500,000 chemical substances from CAS REGISTRY cover areas of community interest, including common and frequently regulated chemicals, and those relevant to high school and undergraduate chemistry classes. This chemical information, curated by our expert scientists, is provided in alignment with our mission as a division of the American Chemical Society. | |
Explanation | The data from CAS Common Chemistry is provided under a CC-BY-NC 4.0 license, unless otherwise stated. | |
Record name | Triethylene glycol dimercaptan | |
Source | ChemIDplus | |
URL | https://pubchem.ncbi.nlm.nih.gov/substance/?source=chemidplus&sourceid=0014970877 | |
Description | ChemIDplus is a free, web search system that provides access to the structure and nomenclature authority files used for the identification of chemical substances cited in National Library of Medicine (NLM) databases, including the TOXNET system. | |
Record name | Triglycol dimercaptan | |
Source | DTP/NCI | |
URL | https://dtp.cancer.gov/dtpstandard/servlet/dwindex?searchtype=NSC&outputformat=html&searchlist=94782 | |
Description | The NCI Development Therapeutics Program (DTP) provides services and resources to the academic and private-sector research communities worldwide to facilitate the discovery and development of new cancer therapeutic agents. | |
Explanation | Unless otherwise indicated, all text within NCI products is free of copyright and may be reused without our permission. Credit the National Cancer Institute as the source. | |
Record name | Ethanethiol, 2,2'-[1,2-ethanediylbis(oxy)]bis- | |
Source | EPA Chemicals under the TSCA | |
URL | https://www.epa.gov/chemicals-under-tsca | |
Description | EPA Chemicals under the Toxic Substances Control Act (TSCA) collection contains information on chemicals and their regulations under TSCA, including non-confidential content from the TSCA Chemical Substance Inventory and Chemical Data Reporting. | |
Record name | 2,2'-[Ethane-1,2-diylbis(oxy)]diethanethiol | |
Source | EPA DSSTox | |
URL | https://comptox.epa.gov/dashboard/DTXSID9044588 | |
Description | DSSTox provides a high quality public chemistry resource for supporting improved predictive toxicology. | |
Record name | 2,2'-[1,2-ethanediylbis(oxy)]bis(ethanethiol) | |
Source | European Chemicals Agency (ECHA) | |
URL | https://echa.europa.eu/substance-information/-/substanceinfo/100.035.480 | |
Description | The European Chemicals Agency (ECHA) is an agency of the European Union which is the driving force among regulatory authorities in implementing the EU's groundbreaking chemicals legislation for the benefit of human health and the environment as well as for innovation and competitiveness. | |
Explanation | Use of the information, documents and data from the ECHA website is subject to the terms and conditions of this Legal Notice, and subject to other binding limitations provided for under applicable law, the information, documents and data made available on the ECHA website may be reproduced, distributed and/or used, totally or in part, for non-commercial purposes provided that ECHA is acknowledged as the source: "Source: European Chemicals Agency, http://echa.europa.eu/". Such acknowledgement must be included in each copy of the material. ECHA permits and encourages organisations and individuals to create links to the ECHA website under the following cumulative conditions: Links can only be made to webpages that provide a link to the Legal Notice page. | |
Record name | TRIETHYLENE GLYCOL DIMERCAPTAN | |
Source | FDA Global Substance Registration System (GSRS) | |
URL | https://gsrs.ncats.nih.gov/ginas/app/beta/substances/83CA1M23NC | |
Description | The FDA Global Substance Registration System (GSRS) enables the efficient and accurate exchange of information on what substances are in regulated products. Instead of relying on names, which vary across regulatory domains, countries, and regions, the GSRS knowledge base makes it possible for substances to be defined by standardized, scientific descriptions. | |
Explanation | Unless otherwise noted, the contents of the FDA website (www.fda.gov), both text and graphics, are not copyrighted. They are in the public domain and may be republished, reprinted and otherwise used freely by anyone without the need to obtain permission from FDA. Credit to the U.S. Food and Drug Administration as the source is appreciated but not required. | |
Retrosynthesis Analysis
AI-Powered Synthesis Planning: Our tool employs the Template_relevance Pistachio, Template_relevance Bkms_metabolic, Template_relevance Pistachio_ringbreaker, Template_relevance Reaxys, Template_relevance Reaxys_biocatalysis model, leveraging a vast database of chemical reactions to predict feasible synthetic routes.
One-Step Synthesis Focus: Specifically designed for one-step synthesis, it provides concise and direct routes for your target compounds, streamlining the synthesis process.
Accurate Predictions: Utilizing the extensive PISTACHIO, BKMS_METABOLIC, PISTACHIO_RINGBREAKER, REAXYS, REAXYS_BIOCATALYSIS database, our tool offers high-accuracy predictions, reflecting the latest in chemical research and data.
Strategy Settings
Precursor scoring | Relevance Heuristic |
---|---|
Min. plausibility | 0.01 |
Model | Template_relevance |
Template Set | Pistachio/Bkms_metabolic/Pistachio_ringbreaker/Reaxys/Reaxys_biocatalysis |
Top-N result to add to graph | 6 |
Feasible Synthetic Routes
Q1: What is the molecular structure and key properties of DODT?
A1: DODT (C6H14O2S2) possesses a linear structure with a molecular weight of 182.3 g/mol. It features two terminal thiol (-SH) groups connected by a flexible ethoxy-ethylene-ethoxy chain. This structure grants DODT unique reactivity and makes it a valuable building block in various chemical reactions.
Q2: How does DODT perform under different conditions, and what are its main applications?
A2: DODT exhibits good stability in a range of organic solvents. Its two thiol groups readily participate in thiol-ene click reactions, making it a highly sought-after reagent for synthesizing functional polymers, bioconjugates, and dendrimers [, ]. Furthermore, DODT has been investigated as a potential non-malodorous substitute for traditional thiol scavengers in Fmoc-based peptide synthesis [, ].
Q3: What challenges are associated with using DODT in peptide synthesis?
A3: While generally effective, DODT can lead to an undesirable alkylation by-product when used as a scavenger during the acidic cleavage of methionine-containing peptides from solid supports []. This side reaction can be mitigated by replacing DODT with ethanedithiol or using methionine sulfoxide as an alternative to methionine in the peptide sequence [].
Q4: Can DODT be utilized in catalytic applications?
A4: While not directly acting as a catalyst, DODT serves as a crucial building block for creating complex molecules with potential catalytic activities. For example, DODT can be incorporated into macrocyclic structures like bis-dithiamacrocycles, which exhibit interesting coordination behaviors with metal ions []. These metal complexes could potentially act as catalysts in various chemical transformations.
Q5: How have computational techniques contributed to our understanding of DODT?
A5: Computational studies have been instrumental in understanding the behavior of polymers synthesized using DODT. For instance, researchers investigated the viscosity properties of cyclic poly(3,6-dioxa-1,8-octanedithiol) (RDODT) in relation to its molecular weight []. These studies shed light on the unique behavior of cyclic polymers compared to their linear counterparts and provided insights into the factors influencing their physical properties.
Q6: How can the stability and solubility of DODT be enhanced for specific applications?
A6: DODT's stability and solubility can be influenced by factors such as pH, temperature, and solvent polarity. While generally stable in organic solvents, its thiol groups are susceptible to oxidation, especially in alkaline environments. Specific formulation strategies, such as incorporating antioxidants or using protective packaging, might be necessary to improve its long-term stability. Further research is required to fully understand and optimize the stability of DODT-containing formulations.
Q7: What are the safety considerations and analytical techniques relevant to DODT?
A7: While DODT offers a less pungent alternative to other thiols, its safety profile and environmental impact require careful consideration. Standard safety regulations for handling and disposal of chemicals should be strictly followed. Analytical techniques like HPLC and mass spectrometry are commonly employed to monitor DODT's purity, stability, and presence in reaction mixtures [, ].
Q8: How is DODT used in the synthesis of polymers?
A8: DODT's ability to participate in thiol-ene click reactions makes it a valuable building block for synthesizing well-defined polymers with tailored properties. For instance, researchers have utilized DODT to create functionalized polyester nanoparticles via controlled intermolecular chain crosslinking []. By adjusting the reaction conditions and incorporating other monomers, diverse polymers with specific applications can be designed.
Q9: Are there any examples of DODT being used to create functional materials?
A9: DODT has shown promise in the development of advanced materials, such as single-ion conducting polymer electrolytes (SIPEs) for lithium-ion batteries []. By incorporating DODT into a poly(vinylidienefluoride) (PVDF) matrix, researchers created a flexible SIPE membrane with high ionic conductivity and excellent mechanical strength []. These properties make it a promising candidate for enhancing the safety and performance of lithium metal batteries.
Disclaimer and Information on In-Vitro Research Products
Please be aware that all articles and product information presented on BenchChem are intended solely for informational purposes. The products available for purchase on BenchChem are specifically designed for in-vitro studies, which are conducted outside of living organisms. In-vitro studies, derived from the Latin term "in glass," involve experiments performed in controlled laboratory settings using cells or tissues. It is important to note that these products are not categorized as medicines or drugs, and they have not received approval from the FDA for the prevention, treatment, or cure of any medical condition, ailment, or disease. We must emphasize that any form of bodily introduction of these products into humans or animals is strictly prohibited by law. It is essential to adhere to these guidelines to ensure compliance with legal and ethical standards in research and experimentation.