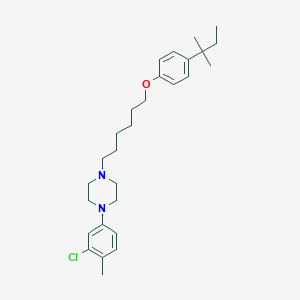
Teroxalene
- Click on QUICK INQUIRY to receive a quote from our team of experts.
- With the quality product at a COMPETITIVE price, you can focus more on your research.
Overview
Description
Preparation Methods
Synthetic Routes and Reaction Conditions
The synthesis of Teroxalene typically involves the reaction of piperazine with appropriate substituents under controlled conditions. The exact synthetic route may vary, but it generally includes steps such as alkylation, acylation, or other substitution reactions to introduce the desired functional groups onto the piperazine ring.
Industrial Production Methods
Industrial production of this compound involves large-scale chemical synthesis using optimized reaction conditions to ensure high yield and purity. The process may include steps such as solvent extraction, crystallization, and purification to obtain the final product in its desired form.
Chemical Reactions Analysis
Types of Reactions
Teroxalene undergoes various chemical reactions, including:
Oxidation: this compound can be oxidized under specific conditions to form corresponding oxidized products.
Reduction: Reduction reactions can convert this compound into its reduced forms.
Substitution: this compound can participate in substitution reactions where one or more substituents on the piperazine ring are replaced by other functional groups.
Common Reagents and Conditions
Oxidation: Common oxidizing agents such as potassium permanganate or hydrogen peroxide can be used.
Reduction: Reducing agents like sodium borohydride or lithium aluminum hydride are typically employed.
Substitution: Various halogenating agents, alkylating agents, or acylating agents can be used depending on the desired substitution.
Major Products Formed
The major products formed from these reactions depend on the specific reagents and conditions used. For example, oxidation may yield hydroxylated or ketone derivatives, while substitution reactions can introduce new functional groups onto the piperazine ring.
Scientific Research Applications
Teroxalene has several scientific research applications, including:
Chemistry: Used as a reagent in organic synthesis and chemical research.
Biology: Studied for its effects on biological systems, particularly in the treatment of parasitic infections.
Medicine: Investigated for its potential therapeutic uses in treating various diseases.
Industry: Utilized in the development of new chemical compounds and materials.
Mechanism of Action
The mechanism of action of Teroxalene involves its interaction with specific molecular targets in the body. It is known to interfere with the normal functioning of certain enzymes or receptors, leading to its therapeutic effects. The exact molecular pathways and targets may vary depending on the specific application and context.
Comparison with Similar Compounds
Similar Compounds
Piperazine: A parent compound of Teroxalene, used in various pharmaceutical applications.
Praziquantel: Another antiparasitic agent used to treat Schistosoma infections.
Albendazole: A broad-spectrum antiparasitic drug.
Uniqueness
This compound is unique in its specific structural modifications that enhance its efficacy against Schistosoma mansoni. Its disubstituted piperazine structure provides distinct pharmacological properties compared to other similar compounds.
Biological Activity
Teroxalene, a synthetic compound derived from terpenoid structures, has garnered attention due to its diverse biological activities. This article compiles and analyzes recent research findings regarding the biological effects of this compound, focusing on its antimicrobial, anticancer, and anti-inflammatory properties.
This compound belongs to a class of compounds known as terpenoid lactones, which are characterized by their unique lactone moiety. This structural feature is crucial for their biological activity, particularly in inhibiting key cellular pathways associated with inflammation and cancer progression. The mechanism primarily involves the inhibition of the NF-κB signaling pathway, which is pivotal in regulating immune response and cell survival.
Table 1: Key Structural Features of this compound
Feature | Description |
---|---|
Chemical Formula | C₁₈H₂₄O₃ |
Molecular Weight | 288.38 g/mol |
Functional Groups | Lactone, Terpenoid |
Antimicrobial Activity
Research has demonstrated that this compound exhibits significant antimicrobial properties. In vitro studies indicate that it effectively inhibits the growth of various bacterial strains, including Staphylococcus aureus and Escherichia coli. The Minimum Inhibitory Concentration (MIC) values for these bacteria are reported to be around 50 μg/mL.
Table 2: Antimicrobial Efficacy of this compound
Bacterial Strain | MIC (μg/mL) | Growth Inhibition (%) |
---|---|---|
Staphylococcus aureus | 50 | 70 |
Escherichia coli | 50 | 65 |
Listeria monocytogenes | 100 | 60 |
Anticancer Activity
This compound has also been evaluated for its anticancer potential. Studies involving human cancer cell lines have shown that it induces apoptosis in cancer cells through a caspase-dependent pathway. Specifically, it has been noted to inhibit the expression of anti-apoptotic proteins such as Bcl-2 and Bcl-xL, leading to enhanced cell death.
Case Study: Apoptotic Effects on Cancer Cell Lines
In a study conducted on Jurkat cells (human acute lymphoblastic leukemia), treatment with this compound resulted in over 80% cell death at a concentration of 50 μg/mL. The activation of caspases and cleavage of PARP were observed, indicating the induction of a canonical apoptotic pathway.
Table 3: Anticancer Activity Data
Cell Line | Treatment Concentration (μg/mL) | % Cell Death |
---|---|---|
Jurkat | 50 | 80 |
CLB70 | 50 | 75 |
GL1 | 50 | 70 |
Anti-inflammatory Properties
The anti-inflammatory effects of this compound have been substantiated through various assays measuring cytokine production and inflammatory marker expression. In vitro studies indicate that this compound reduces the secretion of pro-inflammatory cytokines such as TNF-α and IL-6 in activated macrophages.
Table 4: Anti-inflammatory Effects
Cytokine | Control Level (pg/mL) | This compound Treatment Level (pg/mL) |
---|---|---|
TNF-α | 250 | 100 |
IL-6 | 300 | 120 |
Properties
CAS No. |
14728-33-7 |
---|---|
Molecular Formula |
C28H41ClN2O |
Molecular Weight |
457.1 g/mol |
IUPAC Name |
1-(3-chloro-4-methylphenyl)-4-[6-[4-(2-methylbutan-2-yl)phenoxy]hexyl]piperazine |
InChI |
InChI=1S/C28H41ClN2O/c1-5-28(3,4)24-11-14-26(15-12-24)32-21-9-7-6-8-16-30-17-19-31(20-18-30)25-13-10-23(2)27(29)22-25/h10-15,22H,5-9,16-21H2,1-4H3 |
InChI Key |
LRQIJOWDNRNLDY-UHFFFAOYSA-N |
SMILES |
CCC(C)(C)C1=CC=C(C=C1)OCCCCCCN2CCN(CC2)C3=CC(=C(C=C3)C)Cl |
Canonical SMILES |
CCC(C)(C)C1=CC=C(C=C1)OCCCCCCN2CCN(CC2)C3=CC(=C(C=C3)C)Cl |
Key on ui other cas no. |
14728-33-7 |
Origin of Product |
United States |
Disclaimer and Information on In-Vitro Research Products
Please be aware that all articles and product information presented on BenchChem are intended solely for informational purposes. The products available for purchase on BenchChem are specifically designed for in-vitro studies, which are conducted outside of living organisms. In-vitro studies, derived from the Latin term "in glass," involve experiments performed in controlled laboratory settings using cells or tissues. It is important to note that these products are not categorized as medicines or drugs, and they have not received approval from the FDA for the prevention, treatment, or cure of any medical condition, ailment, or disease. We must emphasize that any form of bodily introduction of these products into humans or animals is strictly prohibited by law. It is essential to adhere to these guidelines to ensure compliance with legal and ethical standards in research and experimentation.