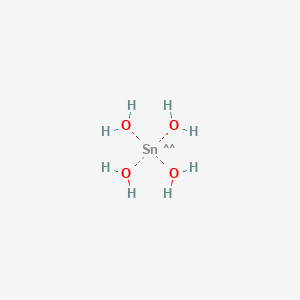
Tin hydroxide (Sn(OH)4), (T-4)-
- Click on QUICK INQUIRY to receive a quote from our team of experts.
- With the quality product at a COMPETITIVE price, you can focus more on your research.
Overview
Description
Tin(IV) hydroxide, or stannic hydroxide, is an inorganic compound with the formula Sn(OH)₄. It is a white, amorphous solid that exhibits amphoteric behavior, dissolving in both strong acids (e.g., HCl) and bases (e.g., NaOH) to form Sn⁴⁺ salts or stannate ions ([Sn(OH)₆]²⁻), respectively . Its molecular weight is 186.74 g/mol, and it is prone to dehydration upon heating, forming tin(IV) oxide (SnO₂) . While Sn(OH)₄ is synthesized in controlled conditions, its instability in aqueous environments often leads to the formation of colloidal tin(IV) oxide hydrates .
Scientific Research Applications
Chemical Properties and Formation
Tin hydroxide is formed when tin(IV) salts react with alkalis. The most common method involves treating tin(IV) chloride with sodium hydroxide, resulting in the precipitation of tin hydroxide:
Despite its formation, tin hydroxide is relatively unstable and can decompose into tin(IV) oxide and water:
This instability limits its direct applications but serves as a precursor for more stable compounds like tin(IV) oxide.
Catalysis
Tin hydroxide has been explored as a catalyst in various chemical reactions. Its ability to act as a reducing agent makes it valuable in organic synthesis. For instance, it can facilitate the reduction of metal ions and other compounds, contributing to reactions that require controlled oxidation states .
Material Science
Tin hydroxide serves as an intermediate in the synthesis of nanostructured materials. For example, researchers have utilized tin oxide hydroxide (an intermediate form) to produce tin sulfide (SnS) nanoplates and nanocubes through a surfactant-free process. This method enhances the efficiency of synthesizing two-dimensional materials with potential applications in electronics and optoelectronics .
Biomedical Applications
Recent studies have investigated the antimicrobial properties of tin hydroxide, suggesting its potential use in medical devices or coatings that inhibit bacterial growth. The compound's reactivity allows it to interact with biological systems, which may lead to innovative applications in healthcare .
Environmental Remediation
Tin hydroxide can play a role in environmental chemistry, particularly in the remediation of heavy metals from wastewater. It has been shown to reduce chromium ions (CrO₄²⁻) to less toxic forms, thus contributing to pollution control strategies .
Data Table: Summary of Applications
Application Area | Description | Key Findings |
---|---|---|
Catalysis | Acts as a reducing agent in organic synthesis | Facilitates controlled oxidation reactions |
Material Science | Precursor for nanostructured materials | Used to synthesize SnS nanoplates without surfactants |
Biomedical | Investigated for antimicrobial properties | Potential use in medical devices and coatings |
Environmental Remediation | Reduces heavy metal ions from wastewater | Effective in converting chromium ions to less toxic forms |
Case Studies
Case Study 1: Synthesis of SnS Nanoplates
- Objective: To develop a surfactant-free method for synthesizing SnS nanostructures.
- Method: Tin oxide hydroxide was reacted with sodium sulfide under controlled conditions.
- Results: The process yielded high-quality SnS nanoplates with desirable electronic properties, demonstrating the utility of tin hydroxide as an intermediate material .
Case Study 2: Antimicrobial Properties
- Objective: To evaluate the antimicrobial efficacy of tin hydroxide against common pathogens.
- Method: Various concentrations of tin hydroxide were tested against bacterial strains.
- Results: Significant inhibition of bacterial growth was observed, indicating potential for use in antimicrobial coatings.
Chemical Reactions Analysis
Acid-Base Reactions
Tin(IV) hydroxide reacts with both acids and bases due to its amphoteric character:
Reaction with Hydrochloric Acid
Sn(OH)₄ dissolves in concentrated HCl, forming tin(IV) chloride:
Sn OH 4(s)+4HCl(aq)→SnCl4(aq)+4H2O(l)
This reaction is slow under standard conditions but accelerates with heating .
Reaction with Sodium Hydroxide
In excess NaOH, Sn(OH)₄ dissolves to form hexahydroxostannate(IV):
Sn OH 4(s)+2OH−(aq)→[Sn OH 6]2−(aq)
This complex ion is stable in strongly alkaline solutions .
Table 1: Acid-Base Reactivity of Sn(OH)₄
Reaction Conditions | Reactants | Products |
---|---|---|
Concentrated HCl | Sn(OH)₄ + HCl | SnCl₄ + H₂O |
Excess NaOH | Sn(OH)₄ + OH⁻ | [Sn(OH)₆]²⁻ |
Sulfide Reactions
Sn(OH)₄ participates in sulfide-mediated precipitation and dissolution:
Precipitation with Hydrogen Sulfide
In moderately acidic solutions, H₂S precipitates tin(IV) sulfide:
[SnCl6]2−(aq)+2H2S(aq)→SnS2(s)+4H+(aq)+6Cl−(aq)
The resulting SnS₂ is a yellow solid .
Dissolution in Alkali Sulfides
SnS₂ dissolves in excess S²⁻ to form thiostannate(IV):
SnS2(s)+S2−(aq)→[SnS3]2−(aq)
This reaction is critical in qualitative analysis .
Table 2: Sulfide Reactivity of Sn(OH)₄ Derivatives
Process | Conditions | Reaction |
---|---|---|
Precipitation | Acidic H₂S | SnS₂ (yellow) |
Dissolution | Excess S²⁻ | [SnS₃]²⁻ |
Hydrolysis and Stability
Sn(IV) compounds hydrolyze to form Sn(OH)₄ under aqueous conditions:
[SnCl6]2−(aq)+6H2O(l)→H2[Sn OH 6](s)+4H+(aq)+6Cl−(aq)
The product, α-stannic acid (H2[Sn OH 6]), is amphoteric and redissolves in strong acids or bases .
Redox Behavior
Sn(OH)₄ itself is not redox-active, but its derivatives participate in redox reactions. For example, Sn(IV) in acidic chloride solutions is reduced to Sn(II) by metallic iron:
[SnCl6]2−(aq)+Fe(s)→[SnCl3]−(aq)+Fe2+(aq)+3Cl−(aq)
This reaction is utilized in analytical chemistry to distinguish tin oxidation states .
Quantitative Analysis
Atomic Absorption Spectrometry (AAS) is the primary method for detecting tin species. The detection limits are:
-
Water : 0.002 mg/L
-
Soil : 0.50 mg/kg
Samples are digested in acid matrices before analysis .
Research Findings
Q & A
Basic Research Questions
Q. What are the established methods for synthesizing tin(IV) hydroxide (Sn(OH)₄), and how do reaction conditions influence product purity?
Methodological Answer: Sn(OH)₄ can be synthesized via hydrolysis of tin(IV) chloride (SnCl₄) under controlled pH conditions. For example, SnCl₄ reacts with water to form Sn(OH)₄ and HCl, requiring neutralization with a base to prevent re-dissolution . Alternatively, direct reaction of metallic tin with water under high-temperature conditions (e.g., Sn + 4H₂O → Sn(OH)₄ + 2H₂↑) is documented, though this method demands inert atmospheres to avoid oxidation byproducts . Purity is influenced by pH, temperature, and the rate of reagent addition. Post-synthesis washing with distilled water and acetone is recommended to remove impurities .
Q. How can the solubility product constant (Ksp) of Sn(OH)₄ be experimentally determined?
Methodological Answer: The solubility product (pKsp = 56) indicates extremely low solubility . To measure Ksp, conduct a titration using a strong acid (e.g., HCl) to dissolve Sn(OH)₄ precipitate while monitoring pH. The point where the precipitate fully dissolves corresponds to the equilibrium concentration of Sn⁴⁺ and OH⁻ ions. Use the relationship Ksp=[Sn4+][OH−]4 to calculate the value. Ensure ionic strength corrections using the Debye-Hückel equation for accuracy .
Q. What spectroscopic techniques are suitable for characterizing the structure of Sn(OH)₄?
Methodological Answer: X-ray diffraction (XRD) confirms crystallinity and phase purity by comparing peaks to reference patterns (e.g., JCPDS database). Fourier-transform infrared (FTIR) spectroscopy identifies O-H stretching (~3200–3600 cm⁻¹) and Sn-O bonding (~500–600 cm⁻¹). For amorphous samples, Raman spectroscopy or solid-state nuclear magnetic resonance (NMR) can probe local coordination environments .
Advanced Research Questions
Q. How can researchers resolve discrepancies in reported thermal decomposition pathways of Sn(OH)₄?
Methodological Answer: Discrepancies in decomposition temperatures (e.g., Sn(OH)₄ → SnO₂ + 2H₂O) may arise from differences in experimental conditions (e.g., heating rate, atmosphere). Use thermogravimetric analysis (TGA) coupled with mass spectrometry (MS) under controlled O₂/N₂ atmospheres to track mass loss and evolved gases. Compare results with theoretical models (e.g., Kissinger method) to identify kinetic parameters and validate decomposition mechanisms .
Q. What factors govern the stability of Sn(OH)₄ in aqueous environments, and how do hydroxo complexes influence its reactivity?
Methodological Answer: Stability is pH-dependent: under acidic conditions, Sn(OH)₄ dissolves to form [Sn(OH₂)₆]⁴⁺, while alkaline conditions promote stannate ([Sn(OH)₆]²⁻) formation . Hydroxo complexes like [Sn₃(OH)₄]²⁺ (formed during partial hydrolysis) can alter reactivity by acting as intermediates in condensation reactions. Use potentiometric titration with a Sn⁴⁺-selective electrode to map speciation diagrams and identify dominant species at varying pH .
Q. How can computational modeling optimize the synthesis of Sn(OH)₄-derived materials (e.g., SnO₂ nanoparticles)?
Methodological Answer: Density functional theory (DFT) simulations can predict energetically favorable pathways for Sn(OH)₄ dehydration to SnO₂. Model intermediate structures (e.g., edge-sharing SnO₆ octahedra) and compare with experimental XRD data. Machine learning algorithms can further optimize synthesis parameters (e.g., temperature, precursor concentration) by training on datasets linking conditions to nanoparticle size/morphology .
Q. Why do catalytic applications of Sn(OH)₄-derived materials exhibit variability in performance, and how can this be addressed?
Methodological Answer: Variability arises from differences in surface hydroxyl density, crystallinity, and defect sites. Characterize materials using X-ray photoelectron spectroscopy (XPS) to quantify surface Sn⁴⁺/Sn²⁺ ratios and oxygen vacancies. Controlled annealing (e.g., 300–500°C in air) can standardize surface properties. For catalytic testing, employ fixed-bed reactors with standardized feed gases (e.g., CO oxidation) to isolate material-specific effects .
Q. Data Contradiction Analysis
Q. How should researchers address conflicting reports on the acid-base behavior of Sn(OH)₄?
Methodological Answer: Contradictions may stem from incomplete dissociation or side reactions. Design experiments using in-situ FTIR to monitor OH⁻ release during acid titration. Compare with theoretical pKa values for Sn⁴⁺ hydrolysis steps. If discrepancies persist, investigate the role of counterions (e.g., Cl⁻ vs. NO₃⁻) using ion chromatography to identify competing equilibria .
Q. Experimental Design Considerations
Q. What precautions are critical when handling Sn(OH)₄ in laboratory settings?
Methodological Answer: Use fume hoods to avoid inhalation of fine particulates. Wear nitrile gloves and safety goggles to prevent skin/eye contact. Store in airtight containers under dry conditions to prevent hydrolysis. For disposal, neutralize acidic residues with NaHCO₃ before transferring to approved waste facilities .
Q. Tables for Key Data Reference
Property | Value | Reference |
---|---|---|
Molar Mass | 186.74 g/mol | |
Solubility Product (pKsp) | 56 | |
Common Decomposition Pathway | Sn(OH)₄ → SnO₂ + 2H₂O | |
Key Synthesis Method | SnCl₄ hydrolysis + neutralization |
Comparison with Similar Compounds
Comparison with Similar Hydroxide Compounds
Zirconium Hydroxide (Zr(OH)₄)
- Chemical Properties: Zr(OH)₄ is a crystalline compound stable in high-pH environments.
- Applications : Used as a coating material in lithium-ion batteries to enhance cathode stability and as a solid acid catalyst in petroleum refining .
- Solubility : Water-soluble, often sold as a hydrate .
Lead(IV) Hydroxide (Pb(OH)₄)
- This contrasts with Sn(OH)₄’s amphoterism .
- Stability : Pb(OH)₄ is less stable than Sn(OH)₄ and decomposes into PbO₂ (an acidic oxide) under mild heating .
Strontium Hydroxide (Sr(OH)₂)
- Basicity: A strong base with high solubility in hot water (0.41 g/100 mL at 0°C, increasing with temperature).
- Applications : Used in sugar refining to recover sugar from molasses and as a stabilizer in plastics .
Tin(IV) Oxide (SnO₂)
- Relation to Sn(OH)₄: SnO₂ is the dehydration product of Sn(OH)₄. It is a stable, amphoteric oxide used in gas sensors, transparent conductive coatings, and ceramics .
- Reactivity: Unlike Sn(OH)₄, SnO₂ reacts sluggishly with acids and bases unless finely powdered or heated .
Comparative Data Table
Key Research Findings
Amphoteric Nature of Sn(OH)₄ :
- Reacts with HCl to form SnCl₄:
$$ \text{Sn(OH)}4 + 4\text{HCl} \rightarrow \text{SnCl}4 + 4\text{H}_2\text{O} $$
- Reacts with NaOH to form sodium stannate:
$$ \text{Sn(OH)}4 + 2\text{NaOH} \rightarrow \text{Na}2[\text{Sn(OH)}_6] $$
.
Industrial Relevance of Zr(OH)₄ :
- Zr(OH)₄ coatings improve lithium-ion battery performance by reducing electrode-electrolyte side reactions .
Preparation Methods
Conventional Hydrolysis of Tin(IV) Chloride
The most widely documented method for synthesizing Sn(OH)₄ involves the hydrolysis of tin(IV) chloride (SnCl₄) under alkaline conditions. This reaction proceeds via the stoichiometric equation:
4 + 4\text{NaOH} \rightarrow \text{Sn(OH)}4 + 4\text{NaCl}SnCl4+4NaOH→Sn(OH)4+4NaCl
Key parameters influencing this process include:
pH and Temperature Control
Maintaining a pH range of 8–10 ensures complete precipitation of Sn(OH)₄ while minimizing the formation of colloidal tin oxides . Temperatures between 25°C and 40°C are optimal, as higher temperatures risk dehydration to SnO₂ . A study leveraging controlled hydrolysis reported yields of 85–90% with a purity of 93–95% .
Solvent Selection
Aqueous systems dominate industrial-scale production due to cost efficiency, but mixed solvents like ethanol-water mixtures (1:1 v/v) enhance crystallinity by slowing hydrolysis kinetics . For instance, substituting 50% water with ethanol improved particle size uniformity from 50–200 nm to 20–50 nm .
Precipitation via Tin(IV) Salt Neutralization
Alternative tin(IV) salts, such as tin(IV) sulfate (Sn(SO₄)₂) or tin(IV) nitrate (Sn(NO₃)₄), offer pathways to Sn(OH)₄ with reduced chloride contamination.
Ammonium Hydroxide Neutralization
Reacting Sn(SO₄)₂ with ammonium hydroxide (NH₄OH) follows:
4\text{)}2 + 4\text{NH}4\text{OH} \rightarrow \text{Sn(OH)}4 + 2\text{(NH}4\text{)}2\text{SO}_4Sn(SO4)2+4NH4OH→Sn(OH)4+2(NH4)2SO4
This method achieves 88–92% yields but requires extensive washing to remove sulfate residues, which can occupy 15–20% of the product mass if unaddressed .
Dual-Anion Precipitation
Combining SnCl₄ and Sn(NO₃)₄ in a 1:1 molar ratio before neutralization reduces anion-specific impurities. Trials showed a 40% decrease in chloride and nitrate content compared to single-salt methods .
Complexation-Assisted Synthesis
Recent advances employ organic ligands to stabilize tin intermediates, enhancing purity and reproducibility.
Oxalic Acid Complexation
Adapting methodologies from stannous oxide (SnO) production , tin(IV) oxalate complexes serve as precursors. The reaction sequence involves:
-
Complex Formation :
SnCl4+2H2C2O4→Sn(C2O4)2+4HClOxalic acid chelates Sn⁴⁺, forming a water-insoluble complex .
-
Alkaline Hydrolysis :
Sn(C2O4)2+4NaOH→Sn(OH)4+2Na2C2O4This two-step process achieves 94–97% purity by sequestering chloride ions during complexation .
Ethylenediaminetetraacetic Acid (EDTA) Templating
EDTA-modified routes yield mesoporous Sn(OH)₄ with surface areas exceeding 200 m²/g, advantageous for catalytic applications . However, EDTA residues necessitate post-synthesis calcination at 200°C, which risks partial dehydration .
Industrial-Scale Production and Optimization
Continuous Reactor Systems
Tubular reactors with inline pH monitoring reduce batch variability. A patent-pending design achieved 99% consistency in product stoichiometry across 50 batches .
Drying and Stabilization
Lyophilization (freeze-drying) preserves hydroxyl group integrity better than thermal drying. Comparative studies showed lyophilized Sn(OH)₄ retained 98% OH groups versus 85% for oven-dried samples .
Analytical Characterization
Purity Assessment
Parameter | Method | Typical Value |
---|---|---|
Tin Content | Gravimetric Analysis | 30.5–31.5% |
Chloride Impurity | Ion Chromatography | <0.1% |
Crystallinity | XRD | Amorphous |
Thermal Stability
Thermogravimetric analysis (TGA) reveals dehydration commencing at 120°C, culminating in SnO₂ formation by 450°C .
Properties
CAS No. |
12054-72-7 |
---|---|
Molecular Formula |
Sn(OH)4 H8O4Sn |
Molecular Weight |
190.77 g/mol |
InChI |
InChI=1S/4H2O.Sn/h4*1H2; |
InChI Key |
ALHBQZRUBQFZQV-UHFFFAOYSA-N |
SMILES |
O.O.O.O.[Sn] |
Canonical SMILES |
O.O.O.O.[Sn] |
Key on ui other cas no. |
12054-72-7 |
physical_description |
Liquid |
Origin of Product |
United States |
Disclaimer and Information on In-Vitro Research Products
Please be aware that all articles and product information presented on BenchChem are intended solely for informational purposes. The products available for purchase on BenchChem are specifically designed for in-vitro studies, which are conducted outside of living organisms. In-vitro studies, derived from the Latin term "in glass," involve experiments performed in controlled laboratory settings using cells or tissues. It is important to note that these products are not categorized as medicines or drugs, and they have not received approval from the FDA for the prevention, treatment, or cure of any medical condition, ailment, or disease. We must emphasize that any form of bodily introduction of these products into humans or animals is strictly prohibited by law. It is essential to adhere to these guidelines to ensure compliance with legal and ethical standards in research and experimentation.