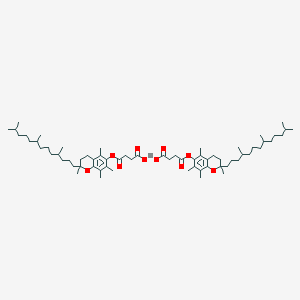
Tocopherol calcium succinate
Overview
Description
Tocopherol calcium succinate is a derivative of tocopherol, which is a form of vitamin E. This compound combines the antioxidant properties of tocopherol with the stability and bioavailability enhancements provided by calcium succinate. Tocopherol is known for its role in protecting cells from oxidative damage, while calcium succinate aids in the compound’s stability and absorption in the body .
Mechanism of Action
Target of Action
Tocopherol calcium succinate, a form of vitamin E, is primarily targeted at the body’s cells, particularly those with highly unsaturated phospholipid bilayers in their cell membranes . These targets play a crucial role in controlling lipid oxidation at the initiation site .
Mode of Action
This compound interacts with its targets by localizing within the cell membrane, providing a means of controlling lipid oxidation . It is believed to undergo a logical de-esterification in the gastrointestinal tract before being subsequently absorbed as free tocopherol . This compound has been shown to inhibit cell growth in vitro, possibly due to its ability to bind to proliferating cells and prevent them from dividing .
Biochemical Pathways
The biosynthesis of tocopherols involves two metabolic pathways: homogentisic acid, an intermediate of degradation of aromatic amino acids, and phytyldiphosphate, which arises from the methylerythritol phosphate pathway . The regulation of tocopherol biosynthesis in photosynthetic organisms occurs at the level of key enzymes including p-hydroxyphenylpyruvate dioxygenase (HPPD), homogentisate phytyltransferase (HPT), tocopherol cyclase (TC), and two methyltransferases .
Pharmacokinetics
This compound is generally believed to undergo a logical de-esterification in the gastrointestinal tract before being subsequently absorbed as free tocopherol . This process is crucial for the compound’s bioavailability and its ability to exert its effects on the body.
Result of Action
The primary result of this compound’s action is its antioxidant effect. As a major lipid-soluble chain-breaking antioxidant, it has the capability to neutralize endogenous free radicals .
Action Environment
The action of this compound can be influenced by various environmental factors. For instance, its biosynthesis changes during plant development and in response to different stresses induced by high-intensity light, drought, high salinity, heavy metals, and chilling . Understanding these influences can help optimize the use of this compound in various applications.
Biochemical Analysis
Biochemical Properties
Tocopherol calcium succinate interacts with various enzymes, proteins, and other biomolecules. It is involved in two metabolic pathways: the degradation of aromatic amino acids, which produces homogentisic acid, and the methylerythritol phosphate pathway, which produces phytyldiphosphate . These interactions are crucial for the biosynthesis of tocopherols .
Cellular Effects
This compound has significant effects on various types of cells and cellular processes. It is known to have pro-apoptotic properties, selectively killing cells with a malignant or transformed phenotype, such as multiple haematopoietic and carcinoma cell lines . It also influences cell function by impacting cell signaling pathways, gene expression, and cellular metabolism .
Molecular Mechanism
The molecular mechanism of action of this compound involves its antioxidant abilities. It prevents the propagation of free radicals in membranes, reacting 1,000 times faster with peroxyl radicals than with polyunsaturated fatty acids . Moreover, it is believed that this compound can elicit anti-cancer and inflammation mediation activities that are unique from the alpha-tocopherol form and other alpha-tocopherol esters .
Temporal Effects in Laboratory Settings
The effects of this compound change over time in laboratory settings. It has been observed that the compound exhibits selective cytotoxicity against tumor cells and very limited or no toxicity toward nonmalignant cells . Information on the product’s stability, degradation, and long-term effects on cellular function is still being researched.
Metabolic Pathways
This compound is involved in the metabolic pathways of homogentisic acid and phytyldiphosphate . It interacts with enzymes and cofactors within these pathways, potentially affecting metabolic flux or metabolite levels.
Transport and Distribution
This compound is transported and distributed within cells and tissues. It is believed that cytosolic binding proteins facilitate the transport of the compound into the mitochondrial and microsomal membranes .
Subcellular Localization
The subcellular localization of this compound is primarily within the highly unsaturated phospholipid bilayer of cell membranes, providing a means of controlling lipid oxidation at the initiation site . This localization is crucial for maintaining oxidative stability of the membrane-bound lipids and preventing damage from reactive oxygen species .
Preparation Methods
Synthetic Routes and Reaction Conditions: The preparation of tocopherol calcium succinate typically involves the esterification of tocopherol with succinic acid, followed by the reaction with a calcium compound. The process can be summarized as follows:
Esterification: Tocopherol is reacted with succinic acid in the presence of a catalyst to form tocopherol succinate.
Salt Formation: The tocopherol succinate is then reacted with a calcium compound, such as calcium hydroxide or calcium carbonate, to form this compound.
Industrial Production Methods: Industrial production of this compound often involves a two-step process:
Initial Esterification: Tocopherol is esterified with succinic acid to form tocopherol succinic acid.
Calcium Salt Formation: The tocopherol succinic acid is then reacted with a calcium compound to form this compound.
Types of Reactions:
Oxidation: this compound can undergo oxidation reactions, where it acts as an antioxidant by donating electrons to neutralize free radicals.
Reduction: The compound can also participate in reduction reactions, particularly in biological systems where it helps in reducing oxidative stress.
Common Reagents and Conditions:
Oxidation: Common oxidizing agents include hydrogen peroxide and oxygen.
Reduction: Reducing agents such as ascorbic acid can be used.
Substitution: Various acids and bases can facilitate substitution reactions.
Major Products Formed:
Oxidation: Tocopherol quinone and other oxidized derivatives.
Reduction: Reduced forms of tocopherol.
Substitution: Various substituted derivatives depending on the reagents used.
Scientific Research Applications
Tocopherol calcium succinate has a wide range of applications in scientific research:
Chemistry: Used as an antioxidant in various chemical reactions to prevent oxidation of sensitive compounds.
Biology: Studied for its role in protecting cells from oxidative damage and its potential in reducing oxidative stress-related diseases.
Medicine: Investigated for its potential in treating conditions related to vitamin E deficiency, cardiovascular diseases, and certain types of cancer.
Industry: Utilized in the food industry as a preservative to enhance the shelf life of products by preventing oxidation
Comparison with Similar Compounds
Alpha-Tocopherol: The most common form of vitamin E, known for its potent antioxidant properties.
Gamma-Tocopherol: Another form of vitamin E with unique antioxidant capabilities.
Tocotrienols: Related compounds with similar antioxidant properties but different chemical structures.
Uniqueness of Tocopherol Calcium Succinate: this compound stands out due to its enhanced stability and bioavailability compared to other forms of tocopherol. The addition of the calcium succinate moiety not only improves its absorption but also provides additional health benefits associated with calcium .
Biological Activity
Tocopherol calcium succinate is a synthetic derivative of vitamin E, specifically designed to enhance its bioavailability and efficacy. This compound exhibits significant biological activities, particularly in antioxidant defense, cellular protection, and potential therapeutic applications in various diseases. This article will explore its biological activity, mechanisms of action, and relevant research findings.
This compound is characterized by its ability to scavenge free radicals and reactive oxygen species (ROS), thereby preventing oxidative damage to cellular components such as lipids, proteins, and DNA. Its primary mechanism involves:
- Antioxidant Activity : this compound acts as an antioxidant by reducing oxidative stress, which is implicated in various diseases including cancer and cardiovascular disorders.
- Cell Signaling Modulation : The compound influences cell signaling pathways associated with inflammation and apoptosis. It modulates the activity of protein kinase C (PKC), which plays a crucial role in cell proliferation and differentiation .
Antioxidant Properties
This compound demonstrates powerful antioxidant properties. It effectively reduces lipid peroxidation and protects against oxidative stress-induced cellular damage. Studies show that it can enhance the intracellular levels of alpha-tocopherol, especially in calcium-deficient environments, thus contributing to cellular health .
Cellular Protection
Research indicates that this compound protects hepatocytes from chemical-induced toxicity. In vitro studies reveal that it provides significant protection against hepatocyte injury under physiological calcium conditions, suggesting its role in maintaining cellular integrity during oxidative stress .
Anti-Cancer Potential
This compound has been investigated for its anti-cancer properties. It has been shown to induce apoptosis in cancer cells while protecting normal cells from adverse effects. Preclinical studies suggest that this compound may enhance the efficacy of conventional cancer therapies such as radiation and chemotherapy .
Research Findings
A variety of studies have explored the biological activity of this compound:
- In Vitro Studies : These studies demonstrate that this compound inhibits the growth of various cancer cell lines while promoting apoptosis. For instance, it has been shown to decrease the proliferation of colon cancer cells significantly .
- In Vivo Studies : Animal models have illustrated that this compound can reduce tumor growth in transgenic mice models of prostate cancer, highlighting its potential as an adjunct therapy in cancer treatment .
Case Studies
- Hepatocyte Protection : A study focusing on isolated hepatocytes revealed that this compound supplementation resulted in higher intracellular alpha-tocopherol levels compared to controls, indicating enhanced antioxidant capacity under low-calcium conditions .
- Cancer Treatment Synergy : In a clinical setting, this compound was administered alongside chemotherapeutic agents, resulting in improved outcomes in tumor reduction compared to chemotherapy alone .
Data Table: Summary of Biological Activities
Properties
IUPAC Name |
calcium;4-oxo-4-[[(2R)-2,5,7,8-tetramethyl-2-[(4R,8R)-4,8,12-trimethyltridecyl]-3,4-dihydrochromen-6-yl]oxy]butanoate | |
---|---|---|
Source | PubChem | |
URL | https://pubchem.ncbi.nlm.nih.gov | |
Description | Data deposited in or computed by PubChem | |
InChI |
InChI=1S/2C33H54O5.Ca/c2*1-22(2)12-9-13-23(3)14-10-15-24(4)16-11-20-33(8)21-19-28-27(7)31(25(5)26(6)32(28)38-33)37-30(36)18-17-29(34)35;/h2*22-24H,9-21H2,1-8H3,(H,34,35);/q;;+2/p-2/t2*23-,24-,33-;/m11./s1 | |
Source | PubChem | |
URL | https://pubchem.ncbi.nlm.nih.gov | |
Description | Data deposited in or computed by PubChem | |
InChI Key |
QEKBRBCVWVLFHH-QAKUKHITSA-L | |
Source | PubChem | |
URL | https://pubchem.ncbi.nlm.nih.gov | |
Description | Data deposited in or computed by PubChem | |
Canonical SMILES |
CC1=C(C(=C(C2=C1OC(CC2)(C)CCCC(C)CCCC(C)CCCC(C)C)C)OC(=O)CCC(=O)[O-])C.CC1=C(C(=C(C2=C1OC(CC2)(C)CCCC(C)CCCC(C)CCCC(C)C)C)OC(=O)CCC(=O)[O-])C.[Ca+2] | |
Source | PubChem | |
URL | https://pubchem.ncbi.nlm.nih.gov | |
Description | Data deposited in or computed by PubChem | |
Isomeric SMILES |
CC1=C(C(=C(C2=C1O[C@](CC2)(C)CCC[C@H](C)CCC[C@H](C)CCCC(C)C)C)OC(=O)CCC(=O)[O-])C.CC1=C(C(=C(C2=C1O[C@](CC2)(C)CCC[C@H](C)CCC[C@H](C)CCCC(C)C)C)OC(=O)CCC(=O)[O-])C.[Ca+2] | |
Source | PubChem | |
URL | https://pubchem.ncbi.nlm.nih.gov | |
Description | Data deposited in or computed by PubChem | |
Molecular Formula |
C66H106CaO10 | |
Source | PubChem | |
URL | https://pubchem.ncbi.nlm.nih.gov | |
Description | Data deposited in or computed by PubChem | |
Molecular Weight |
1099.6 g/mol | |
Source | PubChem | |
URL | https://pubchem.ncbi.nlm.nih.gov | |
Description | Data deposited in or computed by PubChem | |
CAS No. |
14464-85-8, 14638-18-7 | |
Record name | Tocopherol calcium succinate [JAN] | |
Source | ChemIDplus | |
URL | https://pubchem.ncbi.nlm.nih.gov/substance/?source=chemidplus&sourceid=0014464858 | |
Description | ChemIDplus is a free, web search system that provides access to the structure and nomenclature authority files used for the identification of chemical substances cited in National Library of Medicine (NLM) databases, including the TOXNET system. | |
Record name | alpha-Tocopherol calcium succinate, DL- | |
Source | ChemIDplus | |
URL | https://pubchem.ncbi.nlm.nih.gov/substance/?source=chemidplus&sourceid=0014638187 | |
Description | ChemIDplus is a free, web search system that provides access to the structure and nomenclature authority files used for the identification of chemical substances cited in National Library of Medicine (NLM) databases, including the TOXNET system. | |
Record name | .ALPHA.-TOCOPHEROL CALCIUM SUCCINATE, D- | |
Source | FDA Global Substance Registration System (GSRS) | |
URL | https://gsrs.ncats.nih.gov/ginas/app/beta/substances/BVK87L5TNB | |
Description | The FDA Global Substance Registration System (GSRS) enables the efficient and accurate exchange of information on what substances are in regulated products. Instead of relying on names, which vary across regulatory domains, countries, and regions, the GSRS knowledge base makes it possible for substances to be defined by standardized, scientific descriptions. | |
Explanation | Unless otherwise noted, the contents of the FDA website (www.fda.gov), both text and graphics, are not copyrighted. They are in the public domain and may be republished, reprinted and otherwise used freely by anyone without the need to obtain permission from FDA. Credit to the U.S. Food and Drug Administration as the source is appreciated but not required. | |
Record name | .ALPHA.-TOCOPHEROL CALCIUM SUCCINATE, DL- | |
Source | FDA Global Substance Registration System (GSRS) | |
URL | https://gsrs.ncats.nih.gov/ginas/app/beta/substances/H5A1374A6R | |
Description | The FDA Global Substance Registration System (GSRS) enables the efficient and accurate exchange of information on what substances are in regulated products. Instead of relying on names, which vary across regulatory domains, countries, and regions, the GSRS knowledge base makes it possible for substances to be defined by standardized, scientific descriptions. | |
Explanation | Unless otherwise noted, the contents of the FDA website (www.fda.gov), both text and graphics, are not copyrighted. They are in the public domain and may be republished, reprinted and otherwise used freely by anyone without the need to obtain permission from FDA. Credit to the U.S. Food and Drug Administration as the source is appreciated but not required. | |
Retrosynthesis Analysis
AI-Powered Synthesis Planning: Our tool employs the Template_relevance Pistachio, Template_relevance Bkms_metabolic, Template_relevance Pistachio_ringbreaker, Template_relevance Reaxys, Template_relevance Reaxys_biocatalysis model, leveraging a vast database of chemical reactions to predict feasible synthetic routes.
One-Step Synthesis Focus: Specifically designed for one-step synthesis, it provides concise and direct routes for your target compounds, streamlining the synthesis process.
Accurate Predictions: Utilizing the extensive PISTACHIO, BKMS_METABOLIC, PISTACHIO_RINGBREAKER, REAXYS, REAXYS_BIOCATALYSIS database, our tool offers high-accuracy predictions, reflecting the latest in chemical research and data.
Strategy Settings
Precursor scoring | Relevance Heuristic |
---|---|
Min. plausibility | 0.01 |
Model | Template_relevance |
Template Set | Pistachio/Bkms_metabolic/Pistachio_ringbreaker/Reaxys/Reaxys_biocatalysis |
Top-N result to add to graph | 6 |
Feasible Synthetic Routes
Q1: What is the subject of the research paper?
A1: The research paper focuses on the chemical synthesis of tocopherol calcium succinate [].
Q2: Does the paper provide information on the properties or applications of this compound?
A2: Unfortunately, the abstract does not elaborate on the properties, applications, or characterization of this compound. It solely focuses on the synthesis process [].
Disclaimer and Information on In-Vitro Research Products
Please be aware that all articles and product information presented on BenchChem are intended solely for informational purposes. The products available for purchase on BenchChem are specifically designed for in-vitro studies, which are conducted outside of living organisms. In-vitro studies, derived from the Latin term "in glass," involve experiments performed in controlled laboratory settings using cells or tissues. It is important to note that these products are not categorized as medicines or drugs, and they have not received approval from the FDA for the prevention, treatment, or cure of any medical condition, ailment, or disease. We must emphasize that any form of bodily introduction of these products into humans or animals is strictly prohibited by law. It is essential to adhere to these guidelines to ensure compliance with legal and ethical standards in research and experimentation.