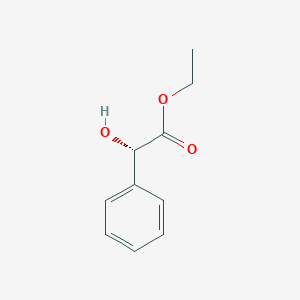
Ethyl (S)-(+)-mandelate
Overview
Description
Ethyl (S)-(+)-mandelate is an organic compound that belongs to the class of mandelic acid derivatives. It is characterized by the presence of an ethyl ester group attached to the mandelic acid backbone. This compound is notable for its chiral nature, with the (S)-(+)-enantiomer being the active form. This compound is widely used in various fields, including organic synthesis, pharmaceuticals, and as a chiral building block in asymmetric synthesis.
Mechanism of Action
Mode of Action
It is known that the compound can be used as a starting material for the preparation of N-benzyl 2-phenylacetamide derivatives, which are known as potent anticonvulsant agents .
Biochemical Pathways
It’s known that ester compounds can undergo hydrolysis, a chemical reaction in which a molecule is split into two parts by the addition of a water molecule . This process could potentially affect various biochemical pathways in the body.
Pharmacokinetics
It’s known that ester compounds can be metabolized in the body through processes such as hydrolysis .
Result of Action
It’s known that the compound can be used as a starting material for the preparation of n-benzyl 2-phenylacetamide derivatives, which are known as potent anticonvulsant agents .
Action Environment
The action of Ethyl (S)-(+)-mandelate can be influenced by various environmental factors. For instance, the pH of the environment can affect the rate of hydrolysis of ester compounds . Additionally, factors such as temperature and the presence of other chemicals can also influence the compound’s action, efficacy, and stability.
Biochemical Analysis
Cellular Effects
It is speculated that this compound may influence cell function, potentially impacting cell signaling pathways, gene expression, and cellular metabolism .
Molecular Mechanism
It is proposed that this compound may exert its effects at the molecular level through binding interactions with biomolecules, enzyme inhibition or activation, and changes in gene expression .
Dosage Effects in Animal Models
Future studies could explore potential threshold effects, as well as any toxic or adverse effects at high doses .
Transport and Distribution
The transport and distribution of Ethyl (S)-(+)-mandelate within cells and tissues are not well characterized. Future studies could explore any transporters or binding proteins that this compound interacts with, as well as any effects on its localization or accumulation .
Preparation Methods
Synthetic Routes and Reaction Conditions: Ethyl (S)-(+)-mandelate can be synthesized through several methods. One common approach involves the esterification of (S)-(+)-mandelic acid with ethanol in the presence of an acid catalyst such as sulfuric acid. The reaction is typically carried out under reflux conditions to ensure complete conversion. Another method involves the use of ethyl chloroformate and (S)-(+)-mandelic acid in the presence of a base such as triethylamine, which facilitates the formation of the ester bond.
Industrial Production Methods: In industrial settings, the production of this compound often involves large-scale esterification processes. These processes utilize continuous flow reactors to maintain optimal reaction conditions and ensure high yields. The use of immobilized catalysts and advanced separation techniques, such as distillation and crystallization, further enhances the efficiency and purity of the final product.
Chemical Reactions Analysis
Types of Reactions: Ethyl (S)-(+)-mandelate undergoes various chemical reactions, including:
Oxidation: It can be oxidized to form this compound oxide using oxidizing agents such as potassium permanganate or chromium trioxide.
Reduction: The compound can be reduced to this compound alcohol using reducing agents like lithium aluminum hydride.
Substitution: this compound can undergo nucleophilic substitution reactions, where the ethyl ester group is replaced by other nucleophiles such as amines or thiols.
Common Reagents and Conditions:
Oxidation: Potassium permanganate in an aqueous medium under acidic conditions.
Reduction: Lithium aluminum hydride in anhydrous ether.
Substitution: Amines or thiols in the presence of a base such as sodium hydroxide.
Major Products Formed:
Oxidation: this compound oxide.
Reduction: this compound alcohol.
Substitution: Corresponding amides or thioesters.
Scientific Research Applications
Ethyl (S)-(+)-mandelate has a wide range of applications in scientific research:
Chemistry: It is used as a chiral building block in asymmetric synthesis, enabling the production of enantiomerically pure compounds.
Biology: The compound serves as a substrate in enzymatic studies to investigate the activity and specificity of esterases and other enzymes.
Medicine: this compound is employed in the synthesis of pharmaceutical intermediates and active pharmaceutical ingredients, particularly in the development of chiral drugs.
Industry: It is used in the production of fine chemicals, flavors, and fragrances, where its chiral properties are essential for achieving the desired sensory attributes.
Comparison with Similar Compounds
Ethyl (S)-(+)-mandelate can be compared with other mandelic acid derivatives, such as:
Mthis compound: Similar in structure but with a methyl ester group instead of an ethyl ester group. It exhibits similar reactivity but may differ in physical properties such as boiling point and solubility.
Ethyl ®-(-)-mandelate: The enantiomer of this compound, which has opposite optical rotation and may exhibit different biological activity and reactivity.
Ethyl (S)-(+)-lactate: Another chiral ester with a similar structure but derived from lactic acid instead of mandelic acid. It is used in similar applications but may have different reactivity and properties.
This compound stands out due to its specific chiral properties and its utility in asymmetric synthesis, making it a valuable compound in various scientific and industrial applications.
Properties
IUPAC Name |
ethyl (2S)-2-hydroxy-2-phenylacetate | |
---|---|---|
Source | PubChem | |
URL | https://pubchem.ncbi.nlm.nih.gov | |
Description | Data deposited in or computed by PubChem | |
InChI |
InChI=1S/C10H12O3/c1-2-13-10(12)9(11)8-6-4-3-5-7-8/h3-7,9,11H,2H2,1H3/t9-/m0/s1 | |
Source | PubChem | |
URL | https://pubchem.ncbi.nlm.nih.gov | |
Description | Data deposited in or computed by PubChem | |
InChI Key |
SAXHIDRUJXPDOD-VIFPVBQESA-N | |
Source | PubChem | |
URL | https://pubchem.ncbi.nlm.nih.gov | |
Description | Data deposited in or computed by PubChem | |
Canonical SMILES |
CCOC(=O)C(C1=CC=CC=C1)O | |
Source | PubChem | |
URL | https://pubchem.ncbi.nlm.nih.gov | |
Description | Data deposited in or computed by PubChem | |
Isomeric SMILES |
CCOC(=O)[C@H](C1=CC=CC=C1)O | |
Source | PubChem | |
URL | https://pubchem.ncbi.nlm.nih.gov | |
Description | Data deposited in or computed by PubChem | |
Molecular Formula |
C10H12O3 | |
Source | PubChem | |
URL | https://pubchem.ncbi.nlm.nih.gov | |
Description | Data deposited in or computed by PubChem | |
Molecular Weight |
180.20 g/mol | |
Source | PubChem | |
URL | https://pubchem.ncbi.nlm.nih.gov | |
Description | Data deposited in or computed by PubChem | |
Synthesis routes and methods I
Procedure details
Synthesis routes and methods II
Procedure details
Synthesis routes and methods III
Procedure details
Retrosynthesis Analysis
AI-Powered Synthesis Planning: Our tool employs the Template_relevance Pistachio, Template_relevance Bkms_metabolic, Template_relevance Pistachio_ringbreaker, Template_relevance Reaxys, Template_relevance Reaxys_biocatalysis model, leveraging a vast database of chemical reactions to predict feasible synthetic routes.
One-Step Synthesis Focus: Specifically designed for one-step synthesis, it provides concise and direct routes for your target compounds, streamlining the synthesis process.
Accurate Predictions: Utilizing the extensive PISTACHIO, BKMS_METABOLIC, PISTACHIO_RINGBREAKER, REAXYS, REAXYS_BIOCATALYSIS database, our tool offers high-accuracy predictions, reflecting the latest in chemical research and data.
Strategy Settings
Precursor scoring | Relevance Heuristic |
---|---|
Min. plausibility | 0.01 |
Model | Template_relevance |
Template Set | Pistachio/Bkms_metabolic/Pistachio_ringbreaker/Reaxys/Reaxys_biocatalysis |
Top-N result to add to graph | 6 |
Feasible Synthetic Routes
Q1: What is the significance of using Ethyl (S)-(+)-mandelate with Candida antarctica lipase?
A1: The research paper [] investigates the use of lipase from Candida antarctica for the biocatalytic aminolysis of this compound. This is significant because enzymatic reactions, like those catalyzed by lipases, offer several advantages over traditional chemical synthesis. These advantages include high selectivity, mild reaction conditions, and reduced environmental impact. The study focuses on understanding the reaction conditions that optimize the yield and selectivity for the desired product, which is crucial for potential applications in pharmaceutical and fine chemical synthesis.
Disclaimer and Information on In-Vitro Research Products
Please be aware that all articles and product information presented on BenchChem are intended solely for informational purposes. The products available for purchase on BenchChem are specifically designed for in-vitro studies, which are conducted outside of living organisms. In-vitro studies, derived from the Latin term "in glass," involve experiments performed in controlled laboratory settings using cells or tissues. It is important to note that these products are not categorized as medicines or drugs, and they have not received approval from the FDA for the prevention, treatment, or cure of any medical condition, ailment, or disease. We must emphasize that any form of bodily introduction of these products into humans or animals is strictly prohibited by law. It is essential to adhere to these guidelines to ensure compliance with legal and ethical standards in research and experimentation.