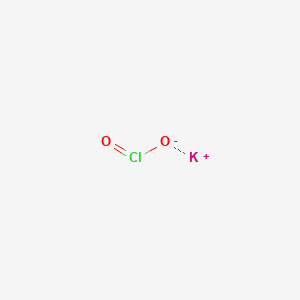
Potassium Chlorite
Overview
Description
Potassium chlorite (KClO₂) is an inorganic compound comprising potassium (K⁺) and chlorite (ClO₂⁻) ions. The chlorite anion contains chlorine in the +3 oxidation state, bonded to two oxygen atoms. This compound is a strong oxidizing agent, typically existing as a stable white to yellowish solid under standard conditions . It is synthesized through reactions between chlorine-based molecules and potassium, similar to other alkali metal chlorites . Its primary applications include water treatment, disinfection, and industrial oxidative processes, though its usage is less common compared to sodium chlorite due to handling and stability considerations .
Preparation Methods
Chemical Synthesis via Chlorine Dioxide Reduction
The most widely recognized method for preparing potassium chlorite involves the reduction of chlorine dioxide (ClO₂) in an alkaline medium. Chlorine dioxide, a potent oxidizing agent, reacts with potassium hydroxide (KOH) in the presence of a reducing agent such as hydrogen peroxide (H₂O₂) or sodium sulfite (Na₂SO₃). The reaction proceeds as follows:
2 + 2\text{KOH} + \text{H}2\text{O}2 \rightarrow 2\text{KClO}2 + 2\text{H}2\text{O} + \text{O}2
This method requires precise control of pH (maintained between 9–11) and temperature (20–40°C) to favor chlorite formation over chlorate or hypochlorite byproducts1. Excess KOH ensures the solution remains basic, while the reducing agent mitigates further oxidation of chlorite to chlorate.
Electrochemical Synthesis
Electrolysis of potassium chloride (KCl) solutions under controlled conditions offers another route to this compound. In this process, a divided electrochemical cell with inert electrodes (e.g., platinum or titanium) is employed. The anode compartment contains a KCl solution, while the cathode compartment holds a dilute KOH solution. Chlorine gas generated at the anode reacts with hydroxyl ions to form hypochlorous acid (HOCl), which disproportionates in basic conditions:
2^- + \text{Cl}^- + 2\text{H}2\text{O}
Subsequent addition of potassium hydroxide precipitates KClO₂. This method demands careful regulation of current density (50–100 mA/cm²) and electrolyte concentration to optimize yield2.
Metathesis Reactions
Metathesis between sodium chlorite (NaClO₂) and potassium salts provides a straightforward pathway to KClO₂. For instance, treating sodium chlorite with potassium nitrate (KNO₃) in aqueous medium facilitates ion exchange:
2 + \text{KNO}3 \rightarrow \text{KClO}2 + \text{NaNO}3
The solubility differences of the products allow selective crystallization of KClO₂. This method is advantageous for large-scale production but requires high-purity starting materials to avoid contamination with chlorate or chloride ions3.
Thermal Decomposition of Potassium Chlorate
Although potassium chlorate (KClO₃) is more stable, controlled thermal decomposition under reduced pressure can yield KClO₂. Heating KClO₃ at 150–200°C in the presence of a catalyst (e.g., manganese dioxide) initiates partial decomposition:
3 \rightarrow \text{KClO}2 + \text{KCl} + 2\text{O}_2
This method is less efficient due to competing reactions but remains a subject of research for specialized applications4.
Analytical Considerations and Quality Control
Quantifying this compound purity necessitates advanced analytical techniques. Ion-specific electrodes or spectrophotometric methods are employed to measure chlorite concentrations, while atomic absorption spectroscopy (AAS) verifies potassium content5. Impurities such as chloride or chlorate are detected via titration with standardized sodium thiosulfate or iodometric methods.
Scientific Research Applications
Chemical Research and Synthesis
Potassium chlorite is extensively utilized as an oxidizing agent in chemical reactions. Its ability to donate oxygen makes it valuable in synthesizing various organic compounds.
- Oxidation Reactions : this compound is used to oxidize alcohols to aldehydes and ketones, and it can also oxidize phenols to quinones. This property is exploited in organic synthesis where selective oxidation is required.
- Synthesis of Chlorine Dioxide : One of the notable applications of this compound is in the production of chlorine dioxide, a potent disinfectant. The reaction involves the acidification of this compound, which results in the release of chlorine dioxide gas.
Biological Applications
In biological research, this compound has been employed to study oxidative stress and its effects on cellular systems.
- Oxidative Stress Studies : Researchers use this compound to induce oxidative stress in cell cultures, allowing for the examination of cellular responses and potential protective mechanisms against oxidative damage.
- Antimicrobial Properties : this compound has been investigated for its antimicrobial properties. It disrupts cellular processes through oxidation, making it effective against a range of bacteria and viruses. This application is particularly relevant in developing disinfectants for healthcare settings.
Industrial Applications
This compound finds numerous applications in various industries due to its bleaching and disinfecting properties.
- Paper and Textile Industries : It is used as a bleaching agent in the paper industry to whiten pulp without damaging fibers. In textiles, this compound helps achieve desired whiteness and colorfastness.
- Water Treatment : this compound is employed as a disinfectant in water treatment facilities. It effectively eliminates pathogens while being less harmful than chlorine.
Medical Applications
In medicine, this compound's properties are harnessed for therapeutic purposes.
- Treatment of Hypokalemia : While potassium chloride is more commonly known for treating low potassium levels (hypokalemia), this compound has been explored for similar uses due to its potassium content.
- Disinfectants : The compound is also used in formulations for disinfecting surfaces and medical equipment, capitalizing on its antimicrobial properties.
Case Study 1: Chlorine Dioxide Production
A study demonstrated the efficacy of this compound in generating chlorine dioxide for water treatment applications. The process involved acidifying a solution of this compound, which led to effective pathogen reduction in treated water samples.
Case Study 2: Antimicrobial Efficacy
Research highlighted the use of this compound as an antimicrobial agent against Escherichia coli and Staphylococcus aureus. The results showed significant reductions in bacterial counts when exposed to this compound solutions, supporting its potential use in healthcare settings.
Data Tables
Application Area | Specific Use | Mechanism/Effect |
---|---|---|
Chemical Research | Oxidation reactions | Oxidizes organic compounds |
Biological Research | Inducing oxidative stress | Disrupts cellular processes |
Industrial | Bleaching agent | Whitens paper/textiles without damage |
Water Treatment | Disinfectant | Eliminates pathogens |
Medical | Antimicrobial disinfectant | Effective against bacteria/viruses |
Mechanism of Action
The mechanism of action of potassium chlorite primarily involves its strong oxidizing properties. It can disrupt cellular processes by oxidizing essential biomolecules such as proteins, lipids, and nucleic acids. This oxidative stress can lead to cell damage and death, making it effective as a disinfectant and antimicrobial agent. The molecular targets include various enzymes and structural proteins within cells .
Comparison with Similar Compounds
Comparison with Similar Potassium-Chlorine-Oxygen Compounds
Chemical Composition and Oxidation States
Compound | Formula | Chlorine Oxidation State | Key Structural Features |
---|---|---|---|
Potassium chloride | KCl | -1 | Simple ionic salt (Cl⁻) |
Potassium hypochlorite | KClO | +1 | Cl–O single bond (OCl⁻) |
Potassium chlorite | KClO₂ | +3 | Cl–O double bond + single bond (ClO₂⁻) |
Potassium chlorate | KClO₃ | +5 | Trigonal pyramidal ClO₃⁻ |
Potassium perchlorate | KClO₄ | +7 | Tetrahedral ClO₄⁻ |
Key Differences :
- Oxidation Strength : Oxidizing power increases with chlorine's oxidation state: KCl < KClO < KClO₂ < KClO₃ < KClO₄ .
- Stability : Thermal stability generally increases with higher oxidation states. For example, KClO decomposes explosively at ~40°C, while KClO₄ is stable up to 400°C . This compound exhibits intermediate stability but is less thermally robust than chlorates or perchlorates.
Reactivity in Redox Reactions
- Iodine Liberation : Chlorite ions (ClO₂⁻) rapidly oxidize iodide (I⁻) to iodine (I₂) in acidic conditions, comparable to bromate (BrO₃⁻) and iodate (IO₃⁻) but faster than chlorate (ClO₃⁻) or perchlorate (ClO₄⁻) . For example, 0.08 g of sodium chlorite reacts completely with 1–5 g KI in sulfuric acid within seconds, whereas chlorate requires >10 minutes .
- Environmental Reduction : Microbial pathways reduce chlorite (ClO₂⁻) to chloride (Cl⁻) via enzymes like chlorite dismutase, whereas perchlorate (ClO₄⁻) reduction involves distinct metabolic pathways .
Geological and Mineralogical Context
- Chlorite Minerals: Naturally occurring chlorites (e.g., Mg-Fe aluminosilicates) are distinct from this compound. Mineral chlorites lack potassium in their structure; any detected potassium arises from contamination by illite or muscovite .
- Reservoir Impacts : Authigenic chlorite coatings in sandstone reservoirs inhibit feldspar overgrowths, unlike potassium-bearing clays like illite .
Biological Activity
Potassium chlorite (KClO2) is an inorganic compound that has garnered attention for its various biological activities, particularly in the fields of medicine and environmental science. This article reviews the biological effects, mechanisms of action, and relevant case studies associated with this compound, supported by diverse research findings.
This compound is a white crystalline solid that is soluble in water. It acts as an oxidizing agent and is often used in disinfection processes due to its ability to release chlorine dioxide (ClO2) when dissolved in water. The biological activity of this compound can be attributed to its oxidative properties, which can affect cellular functions and microbial viability.
- Oxidative Stress Induction : this compound can induce oxidative stress in cells, leading to damage in cellular components such as lipids, proteins, and DNA. This mechanism can be beneficial in antimicrobial applications but may also pose risks to human health if misused.
- Cellular Signaling Modulation : Research indicates that this compound may influence ion channels, particularly potassium and chloride channels, affecting cellular excitability and signaling pathways .
Antimicrobial Properties
This compound demonstrates significant antimicrobial activity. It has been studied for its effectiveness against various pathogens, including bacteria and fungi. The compound's ability to disrupt microbial cell membranes through oxidative damage is a key factor in its efficacy.
Case Study: Antifungal Activity
A study investigated the antifungal effects of this compound against Candida albicans. The results indicated that at sub-minimal inhibitory concentrations (sub-MIC), this compound enhanced the antifungal activity of silymarin by modulating ion channel activity . This suggests potential applications in treating fungal infections when combined with other therapeutic agents.
Clinical Implications and Toxicity
While this compound has potential therapeutic uses, it also poses health risks when misused or ingested inappropriately.
Toxicological Concerns
- Case of Acute Poisoning : A notable case involved a patient who ingested potassium chlorate (KClO3), a related compound, leading to acute toxicity characterized by renal failure and severe systemic effects . Although this case specifically addresses potassium chlorate, it highlights the potential dangers associated with chlorate compounds.
- Chronic Exposure Risks : Chronic ingestion of potassium chlorate has been linked to interstitial nephritis and other renal issues . This raises concerns about the long-term safety of compounds that release chlorine dioxide or similar oxidative species.
Comparative Studies on Potassium Compounds
To better understand the biological effects of this compound compared to other potassium salts, several studies have been conducted:
Chemical Reactions Analysis
Salt Metathesis with Sodium Chlorate
The dominant industrial production method involves reacting sodium chlorate with potassium chloride:
This reaction leverages the low solubility of KClO₃ in water, which drives product precipitation (Le Chatelier’s principle) .
Electrolysis of Potassium Chloride
Direct electrolysis of KCl in aqueous solutions generates potassium chlorate via intermediate chlorine and hydroxide reactions:
This method is less common due to the efficiency of the metathesis route .
Decomposition Reactions
Potassium chlorate decomposes under heat, with products varying based on catalysts and temperature:
Catalytic Decomposition (MnO₂)
In the presence of manganese dioxide (MnO₂), it decomposes to oxygen and potassium chloride:
This reaction is critical in oxygen generators and fireworks .
Thermal Decomposition Without Catalyst
Heating KClO₃ above 400°C produces potassium perchlorate and KCl:
Further heating decomposes KClO₄:
These reactions require precise temperature control to avoid explosive side reactions .
Reaction with Red Phosphorus (Shock-Sensitive Explosive)
Mixing KClO₃ with red phosphorus creates a shock-sensitive explosive mixture. The reaction is highly exothermic:
Substance | ΔG (kJ/mol) |
---|---|
P₄ | -12.1 |
P₄O₁₀ | -2697.7 |
KClO₃ | -296.25 |
KCl | -408.77 |
Total ΔG | -9182 |
This reaction’s large negative ΔG highlights its explosive potential, necessitating extreme caution .
Reaction with Sulfuric Acid
KClO₃ reacts with concentrated sulfuric acid to form chloric acid (HClO₃), a potent oxidizer:
This reaction is utilized in laboratory oxidations .
Chemical Oxygen Generators
In aircraft and submarines, KClO₃ decomposes catalytically to provide emergency oxygen:
Notably, impurities or organic contaminants (e.g., grease) can trigger violent explosions .
Q & A
Basic Research Questions
Q. What analytical methods are recommended for quantifying potassium chlorite in complex mixtures?
- Methodology :
- Ion Chromatography (IC) : Use anion-exchange columns (e.g., Dionex IonPac™ AS19) to separate chlorite (ClO₂⁻) from interfering anions like chloride (Cl⁻) and sulfate (SO₄²⁻). Calibrate with certified reference standards and validate detection limits via spiked recovery experiments .
- Titration : Employ redox titration with potassium permanganate (KMnO₄) in acidic media, monitoring endpoint colorimetrically. Pre-treat samples to remove oxidizable impurities (e.g., organic matter) to avoid false positives .
Q. How should thermal decomposition experiments of this compound be designed to ensure reproducibility?
- Experimental Protocol :
- Use controlled heating ramps (e.g., 2–5°C/min) in inert atmospheres to minimize side reactions. Record mass loss via thermogravimetric analysis (TGA) and correlate with evolved gas analysis (e.g., O₂ detection via gas chromatography) .
- Repeat heating cycles (e.g., two consecutive heats) to confirm complete decomposition and rule out residual intermediates .
Advanced Research Questions
Q. How can researchers resolve contradictions in reported decomposition kinetics of this compound under varying humidity conditions?
- Conflict Analysis :
- Controlled Humidity Chambers : Replicate conflicting studies under standardized humidity levels (e.g., 30% vs. 70% RH) while monitoring reaction rates via in situ Raman spectroscopy to detect intermediate phases (e.g., KClO₃ formation) .
- Statistical Validation : Apply multivariate regression to isolate humidity’s effect from temperature and pressure variables. Use Akaike Information Criterion (AIC) to compare competing kinetic models .
Q. What methodologies are available for assessing the purity of this compound in synthesized samples?
- Multi-Technique Approach :
- X-Ray Diffraction (XRD) : Identify crystalline impurities (e.g., KCl or unreacted KClO₃) by comparing diffraction patterns with ICDD database entries .
- Ion-Specific Electrodes : Quantify chloride (Cl⁻) contamination using Ag/AgCl electrodes, calibrated against NIST-traceable standards .
Q. How can researchers validate conflicting reports on this compound’s oxidative stability in aqueous solutions?
- Controlled Replication :
- Prepare solutions under nitrogen purge to exclude dissolved oxygen. Monitor pH and redox potential (Eh) continuously using microelectrodes .
- Compare degradation rates under UV light vs. dark conditions to assess photolytic contributions .
Q. Methodological Best Practices
Q. What safety protocols are critical when handling this compound in laboratory settings?
- Exposure Mitigation :
- Use fume hoods for all procedures involving chlorite powders or aerosols. Adhere to OSHA guidelines for respiratory protection (N95 masks) and glove compatibility (nitrile > latex) .
- Store chlorite separately from reducing agents (e.g., organic solvents) to prevent accidental combustion .
Q. How should researchers document experimental procedures for reproducibility in this compound studies?
- Data Reporting Standards :
Properties
IUPAC Name |
potassium;chlorite | |
---|---|---|
Source | PubChem | |
URL | https://pubchem.ncbi.nlm.nih.gov | |
Description | Data deposited in or computed by PubChem | |
InChI |
InChI=1S/ClHO2.K/c2-1-3;/h(H,2,3);/q;+1/p-1 | |
Source | PubChem | |
URL | https://pubchem.ncbi.nlm.nih.gov | |
Description | Data deposited in or computed by PubChem | |
InChI Key |
VISKNDGJUCDNMS-UHFFFAOYSA-M | |
Source | PubChem | |
URL | https://pubchem.ncbi.nlm.nih.gov | |
Description | Data deposited in or computed by PubChem | |
Canonical SMILES |
[O-]Cl=O.[K+] | |
Source | PubChem | |
URL | https://pubchem.ncbi.nlm.nih.gov | |
Description | Data deposited in or computed by PubChem | |
Molecular Formula |
KClO2, ClKO2 | |
Record name | potassium chlorite | |
Source | Wikipedia | |
URL | https://en.wikipedia.org/wiki/Dictionary_of_chemical_formulas | |
Description | Chemical information link to Wikipedia. | |
Source | PubChem | |
URL | https://pubchem.ncbi.nlm.nih.gov | |
Description | Data deposited in or computed by PubChem | |
Related CAS |
13898-47-0 (Parent) | |
Record name | Potassium chlorite | |
Source | ChemIDplus | |
URL | https://pubchem.ncbi.nlm.nih.gov/substance/?source=chemidplus&sourceid=0014314273 | |
Description | ChemIDplus is a free, web search system that provides access to the structure and nomenclature authority files used for the identification of chemical substances cited in National Library of Medicine (NLM) databases, including the TOXNET system. | |
DSSTOX Substance ID |
DTXSID90931758 | |
Record name | Potassium chlorite | |
Source | EPA DSSTox | |
URL | https://comptox.epa.gov/dashboard/DTXSID90931758 | |
Description | DSSTox provides a high quality public chemistry resource for supporting improved predictive toxicology. | |
Molecular Weight |
106.55 g/mol | |
Source | PubChem | |
URL | https://pubchem.ncbi.nlm.nih.gov | |
Description | Data deposited in or computed by PubChem | |
CAS No. |
14314-27-3 | |
Record name | Potassium chlorite | |
Source | ChemIDplus | |
URL | https://pubchem.ncbi.nlm.nih.gov/substance/?source=chemidplus&sourceid=0014314273 | |
Description | ChemIDplus is a free, web search system that provides access to the structure and nomenclature authority files used for the identification of chemical substances cited in National Library of Medicine (NLM) databases, including the TOXNET system. | |
Record name | Potassium chlorite | |
Source | EPA DSSTox | |
URL | https://comptox.epa.gov/dashboard/DTXSID90931758 | |
Description | DSSTox provides a high quality public chemistry resource for supporting improved predictive toxicology. | |
Record name | POTASSIUM CHLORITE | |
Source | FDA Global Substance Registration System (GSRS) | |
URL | https://gsrs.ncats.nih.gov/ginas/app/beta/substances/71K32L1LFJ | |
Description | The FDA Global Substance Registration System (GSRS) enables the efficient and accurate exchange of information on what substances are in regulated products. Instead of relying on names, which vary across regulatory domains, countries, and regions, the GSRS knowledge base makes it possible for substances to be defined by standardized, scientific descriptions. | |
Explanation | Unless otherwise noted, the contents of the FDA website (www.fda.gov), both text and graphics, are not copyrighted. They are in the public domain and may be republished, reprinted and otherwise used freely by anyone without the need to obtain permission from FDA. Credit to the U.S. Food and Drug Administration as the source is appreciated but not required. | |
Retrosynthesis Analysis
AI-Powered Synthesis Planning: Our tool employs the Template_relevance Pistachio, Template_relevance Bkms_metabolic, Template_relevance Pistachio_ringbreaker, Template_relevance Reaxys, Template_relevance Reaxys_biocatalysis model, leveraging a vast database of chemical reactions to predict feasible synthetic routes.
One-Step Synthesis Focus: Specifically designed for one-step synthesis, it provides concise and direct routes for your target compounds, streamlining the synthesis process.
Accurate Predictions: Utilizing the extensive PISTACHIO, BKMS_METABOLIC, PISTACHIO_RINGBREAKER, REAXYS, REAXYS_BIOCATALYSIS database, our tool offers high-accuracy predictions, reflecting the latest in chemical research and data.
Strategy Settings
Precursor scoring | Relevance Heuristic |
---|---|
Min. plausibility | 0.01 |
Model | Template_relevance |
Template Set | Pistachio/Bkms_metabolic/Pistachio_ringbreaker/Reaxys/Reaxys_biocatalysis |
Top-N result to add to graph | 6 |
Feasible Synthetic Routes
Disclaimer and Information on In-Vitro Research Products
Please be aware that all articles and product information presented on BenchChem are intended solely for informational purposes. The products available for purchase on BenchChem are specifically designed for in-vitro studies, which are conducted outside of living organisms. In-vitro studies, derived from the Latin term "in glass," involve experiments performed in controlled laboratory settings using cells or tissues. It is important to note that these products are not categorized as medicines or drugs, and they have not received approval from the FDA for the prevention, treatment, or cure of any medical condition, ailment, or disease. We must emphasize that any form of bodily introduction of these products into humans or animals is strictly prohibited by law. It is essential to adhere to these guidelines to ensure compliance with legal and ethical standards in research and experimentation.