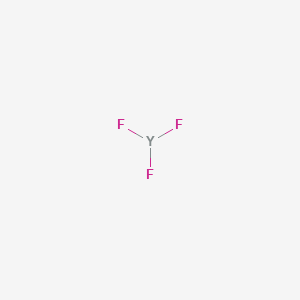
Yttrium fluoride
- Click on QUICK INQUIRY to receive a quote from our team of experts.
- With the quality product at a COMPETITIVE price, you can focus more on your research.
Overview
Description
Yttrium fluoride (YF₃) is an inorganic compound with the molecular formula YF₃, characterized by its orthorhombic crystal structure and high melting point of 1,387°C . It is synthesized via hydrofluorination of yttrium precursors (e.g., yttrium oxide, yttrium hydroxide) using anhydrous hydrogen fluoride gas, with optimized conditions ensuring low oxygen content . YF₃ exhibits broad optical transparency across ultraviolet (UV), visible, and infrared (IR) spectra, making it invaluable in optical coatings, laser systems, and electronic components . Its chemical inertness, high thermal stability, and low solubility in water (<0.01 g/100 mL at 20°C) further enhance its utility in harsh environments .
Preparation Methods
Synthetic Routes and Reaction Conditions: Yttrium fluoride can be synthesized through several methods:
Reaction with Hydrofluoric Acid: One common method involves reacting yttrium hydroxide with hydrofluoric acid: [ Y(OH)_3 + 3HF \rightarrow YF_3 + 3H_2O ]
Direct Fluorination: Another method is the direct fluorination of yttrium oxide using fluorine gas at elevated temperatures: [ Y_2O_3 + 6F_2 \rightarrow 2YF_3 + 3O_2 ]
Industrial Production Methods: In industrial settings, this compound is often produced by passing anhydrous hydrofluoric acid gas over yttrium oxide at intermediate temperatures ranging from 200°C to 800°C . This method ensures high purity and efficient production of this compound.
Chemical Reactions Analysis
Types of Reactions: Yttrium fluoride primarily undergoes substitution reactions due to its stability and resistance to oxidation and reduction. Some common reactions include:
Reaction with Alkali Metals: this compound reacts with alkali metals to form corresponding yttrium compounds and alkali metal fluorides.
Reaction with Acids: It reacts with strong acids, except hydrofluoric acid, to form soluble yttrium salts.
Common Reagents and Conditions:
Alkali Metals: Sodium or potassium can be used in substitution reactions.
Strong Acids: Hydrochloric acid or sulfuric acid can be used to convert this compound into soluble yttrium salts.
Major Products:
Yttrium Chloride: Formed by reacting this compound with hydrochloric acid.
Yttrium Sulfate: Formed by reacting this compound with sulfuric acid.
Scientific Research Applications
Optical Coatings
Yttrium fluoride is widely used in the production of optical coatings, especially for infrared (IR) and ultraviolet (UV) applications. Its high melting point and low refractive index make it an ideal candidate for thin film coatings on lenses and mirrors. This compound exhibits excellent transmissive properties across a broad spectrum, ranging from UV to near-infrared wavelengths, which is crucial for high-performance optical devices .
Key Properties:
- Refractive Index : Approximately 1.51 at 500 nm.
- Transparency Range : Effective from 193 nm (UV) to 14,000 nm (IR).
Application Examples:
- UV Coatings : Used in applications requiring low oxygen environments and high purity to ensure superior quality thin films .
- IR Coatings : Critical for industries where high-performance optical components are essential .
Antibacterial Applications
Recent studies have highlighted the antibacterial and antibiofilm properties of this compound nanoparticles. Research indicates that these nanoparticles can effectively inhibit bacterial growth, particularly against pathogens such as Escherichia coli and Staphylococcus aureus. The antimicrobial activity is influenced by the size of the nanoparticles, with smaller sizes exhibiting enhanced efficacy .
Case Study:
In a study involving catheter surfaces modified with this compound nanoparticles, significant reductions in bacterial colonization were observed compared to uncoated surfaces. This suggests potential applications in medical devices where biofilm formation poses a risk for infections .
Metallurgical Applications
This compound serves as an additive in metallurgy to produce high-strength alloys. Its ability to improve the mechanical properties of metals makes it valuable in manufacturing processes that require enhanced durability and resistance to corrosion .
Benefits:
- High Melting Point : Suitable for high-temperature applications.
- Corrosion Resistance : Enhances the longevity and performance of metal products.
Nanotechnology
The synthesis of this compound nanoparticles through sonochemical methods has opened new avenues in nanotechnology. These nanoparticles are characterized by their unique crystallinity and morphology, which can be tailored for specific applications. Their low solubility contributes to their potential as antimicrobial agents in various medical applications .
Mechanism of Action
The mechanism of action of yttrium fluoride in various applications is primarily based on its chemical stability and optical properties. In biomedical imaging, for example, this compound nanoparticles enhance contrast due to their high refractive index and ability to emit light under specific conditions . In plasma etching, this compound films protect surfaces from erosion by forming a stable, inert layer that resists chemical attack .
Comparison with Similar Compounds
Key Physical and Chemical Properties of YF₃
Property | Value | Reference |
---|---|---|
Molecular Weight | 145.90 g/mol | |
Melting Point | 1,387°C | |
Density | 4.01 g/cm³ | |
Crystal Structure | Orthorhombic | |
Solubility in Water | Insoluble | |
Solubility in Acids | Slightly soluble |
Yttrium fluoride is often compared to other rare-earth fluorides, oxides, and alkaline-earth fluorides due to overlapping applications in optics, metallurgy, and coatings. Below is a detailed analysis:
This compound vs. Yttrium Oxide (Y₂O₃)
- Plasma Erosion Resistance : YF₃ coatings demonstrate superior resistance to fluorocarbon plasma erosion compared to Y₂O₃, minimizing particle contamination in semiconductor manufacturing .
- Optical Properties : YF₃ has broader transparency in UV-IR ranges, whereas Y₂O₃ is primarily used in visible-light applications due to its high refractive index (1.93 vs. YF₃’s 1.5) .
- Thermal Stability : Y₂O₃ has a higher melting point (2,425°C) but lower thermal shock resistance in fluoride-rich environments .
This compound vs. Scandium Fluoride (ScF₃)
- Chemical Behavior : Both form passive layers in water, but ScF₃ is more reactive with acids due to scandium’s smaller ionic radius .
- Applications : ScF₃ is used in high-intensity lamps and catalysts, while YF₃ dominates in optical coatings and antibacterial composites .
This compound vs. Calcium Fluoride (CaF₂)
- Metallurgical Use : CaF₂ is a common flux in steelmaking, whereas YF₃ is critical for synthesizing Mg-Zn-Y master alloys, achieving yttrium yields >99% at 700°C .
- Optical Performance : CaF₂ has superior IR transparency, but YF₃’s lower refractive index reduces light scattering in multilayer coatings .
Comparative Data Table
Research Findings and Industrial Relevance
Antibacterial Activity
YF₃ nanoparticles (1–5 wt%) in orthodontic resins inhibit Streptococcus mutans growth, reducing enamel demineralization without compromising adhesion strength .
Biological Activity
Yttrium fluoride (YF₃) has garnered attention due to its unique properties and potential applications, particularly in the biomedical field. This article explores the biological activity of this compound, focusing on its antimicrobial properties, mechanisms of action, and implications for medical applications.
Overview of this compound
This compound is a rare earth metal fluoride that exhibits low solubility in water, which is significant for its biological applications. The compound can be synthesized into nanoparticles (YF₃ NPs), which have been shown to enhance its biological activity compared to bulk materials.
Efficacy Against Bacterial Pathogens
This compound nanoparticles have demonstrated notable antibacterial activity against common pathogens such as Escherichia coli and Staphylococcus aureus. Research indicates that the antimicrobial effectiveness is size-dependent, with smaller nanoparticles exhibiting greater activity. In a study, YF₃ NPs showed minimum inhibitory concentrations (MICs) in the microgram per milliliter range, suggesting their potential as effective antimicrobial agents .
Table 1: Antimicrobial Activity of this compound Nanoparticles
Bacterial Strain | Minimum Inhibitory Concentration (MIC) |
---|---|
Escherichia coli | μg/mL range |
Staphylococcus aureus | μg/mL range |
The antimicrobial mechanism of this compound is believed to involve disruption of bacterial cell membranes, leading to increased permeability and eventual cell lysis. This effect is enhanced by the nanoparticle's high surface area-to-volume ratio, which facilitates greater interaction with bacterial cells .
Antibiofilm Properties
Biofilm formation on medical devices is a significant challenge in clinical settings. YF₃ NPs have been evaluated for their ability to inhibit biofilm formation on surfaces such as catheters. In studies, catheters coated with YF₃ NPs showed a significant reduction in bacterial colonization compared to uncoated controls. This property is crucial for preventing infections associated with medical implants .
Case Study: Catheter Coating with YF₃ NPs
- Objective : To evaluate the effectiveness of YF₃ NP-coated catheters in reducing bacterial biofilm formation.
- Methodology : Catheters were modified with a homogeneous layer of YF₃ NPs using a one-step synthesis process.
- Results : The NP-coated catheters exhibited significantly lower bacterial colonization rates compared to uncoated catheters, highlighting their potential for use in infection prevention strategies .
Synthesis and Characterization of YF₃ Nanoparticles
The synthesis of this compound nanoparticles typically involves sonochemical methods that produce needle-shaped particles characterized by scanning electron microscopy (SEM) and X-ray diffraction. These methods allow for precise control over particle size and morphology, which are critical factors influencing their biological activity .
Q & A
Basic Research Questions
Q. What are the primary synthesis methods for high-purity YF₃ crystals, and how do process parameters influence crystal quality?
YF₃ synthesis typically employs hydrothermal methods or solid-state reactions . Hydrothermal synthesis involves reacting yttrium oxide (Y₂O₃) with hydrofluoric acid (HF) under controlled temperature (150–250°C) and pressure, producing crystals with 99.9–99.99% purity . Key parameters include:
- Temperature gradients : Affects crystal growth rate and defect density.
- Precursor purity : Impurities >0.1% introduce lattice distortions, reducing optical transparency .
- pH control : Neutral or slightly acidic conditions minimize hydrolysis byproducts.
Q. How do researchers characterize YF₃’s optical properties, and what instrumentation is critical for reproducibility?
Standard characterization includes:
- UV-Vis-NIR spectroscopy : Measures transmittance (85–92% in 200–1500 nm range) and identifies absorption bands linked to rare-earth dopants (e.g., Yb³⁺, Er³⁺) .
- X-ray diffraction (XRD) : Confirms orthorhombic phase purity (PDF# 01-070-1935) .
- Raman spectroscopy : Detects vibrational modes (e.g., 320 cm⁻¹ for Y–F stretching) to assess structural defects .
Reproducibility tip: Calibrate instruments using certified reference materials (CRMs) for fluoride crystals .
Q. What are the key applications of YF₃ in photonics, and how do dopants modify its performance?
YF₃ serves as a host matrix for upconversion nanoparticles (UCNPs) and laser gain media . For example:
- Ho³⁺-doped YF₃ : Emits at 545 nm under 980 nm excitation, used in bioimaging .
- Yb³⁺/Er³⁺ co-doping : Enhances luminescence quantum yield (up to 12%) via energy transfer .
Experimental design: Optimize dopant concentration (0.5–5 mol%) to balance emission intensity and lattice strain .
Advanced Research Questions
Q. How can researchers resolve contradictions in fluoride ion (F⁻) quantification data across studies?
Discrepancies often arise from:
- Sample matrix interference : Aluminum or iron ions >1% bind F⁻, reducing detection accuracy .
- Methodological bias : Compare ion-selective electrode (ISE), ion chromatography (IC), and synchrotron-based XAS results for cross-validation .
Case study: ISE measurements of YF₃ solubility in aqueous media vary by ±15% due to lanthanum nitrate titration endpoint ambiguity .
Q. What strategies mitigate defect formation during YF₃ crystal growth for high-power laser applications?
Defects (e.g., vacancies, dislocations) reduce laser damage thresholds. Solutions include:
- Melt purification : Remove oxygen impurities via reactive atmosphere processing (e.g., CF₄ gas) .
- Interface control : Maintain convex solid-liquid interfaces during Czochralski growth to minimize stress .
- Post-growth annealing : Heal point defects at 600°C under inert gas flow .
Q. How do interfacial properties of YF₃ influence its performance in multilayer optical coatings?
YF₃’s low refractive index (1.5 at 550 nm) makes it ideal for anti-reflective coatings. Challenges include:
- Adhesion failure : Use atomic layer deposition (ALD) to enhance YF₃/Si interface bonding .
- Stress accumulation : Monitor residual stress via in-situ XRD during sputtering (<300 MPa acceptable) .
Q. Data Contradiction Analysis
Q. Why do reported bandgap values for YF₃ vary between eV and eV?
Variations stem from:
- Measurement technique : UV-Vis extrapolation vs. spectroscopic ellipsometry.
- Surface oxidation : YF₃ films exposed to air form YOF surface layers, altering optical properties .
Recommendation: Conduct measurements in ultrahigh vacuum (UHV) to minimize surface contamination .
Q. How to reconcile conflicting solubility data for YF₃ in acidic vs. alkaline environments?
- In 1M HNO₃ : Solubility = 0.12 g/L (25°C) due to Y³⁺ hydration .
- In NH₄OH : Forms soluble [YF₆]³⁻ complexes, increasing solubility to 0.45 g/L .
Methodological note: Use ICP-MS with ¹⁸⁹Y isotope tracking to distinguish dissolution mechanisms .
Q. Research Design Tables
Table 1. Purity Grades and Applications of YF₃
Purity (%) | Application | Key Characterization Methods |
---|---|---|
99.9 | Optical coatings | XRD, AFM, Ellipsometry |
99.99 | Laser crystals | PL spectroscopy, TEM |
99.999 | Quantum dots | SAED, XPS |
Table 2. Common Dopants in YF₃
Dopant | Concentration (mol%) | Emission Wavelength (nm) | Application |
---|---|---|---|
Yb³⁺ | 2–5 | 980 (absorption) | NIR lasers |
Er³⁺ | 1–3 | 545, 660 | Bioimaging |
Ho³⁺ | 0.5–2 | 540, 750 | Temperature sensing |
Q. Methodological Guidelines
Properties
CAS No. |
13709-49-4 |
---|---|
Molecular Formula |
F3Y |
Molecular Weight |
145.90105 g/mol |
IUPAC Name |
yttrium(3+);trifluoride |
InChI |
InChI=1S/3FH.Y/h3*1H;/q;;;+3/p-3 |
InChI Key |
RBORBHYCVONNJH-UHFFFAOYSA-K |
SMILES |
F[Y](F)F |
Canonical SMILES |
[F-].[F-].[F-].[Y+3] |
Key on ui other cas no. |
13709-49-4 |
Pictograms |
Irritant |
Origin of Product |
United States |
Disclaimer and Information on In-Vitro Research Products
Please be aware that all articles and product information presented on BenchChem are intended solely for informational purposes. The products available for purchase on BenchChem are specifically designed for in-vitro studies, which are conducted outside of living organisms. In-vitro studies, derived from the Latin term "in glass," involve experiments performed in controlled laboratory settings using cells or tissues. It is important to note that these products are not categorized as medicines or drugs, and they have not received approval from the FDA for the prevention, treatment, or cure of any medical condition, ailment, or disease. We must emphasize that any form of bodily introduction of these products into humans or animals is strictly prohibited by law. It is essential to adhere to these guidelines to ensure compliance with legal and ethical standards in research and experimentation.