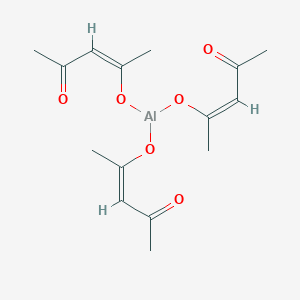
Aluminium acetylacetonate
- Click on QUICK INQUIRY to receive a quote from our team of experts.
- With the quality product at a COMPETITIVE price, you can focus more on your research.
Overview
Description
Aluminium acetylacetonate is a coordination complex derived from the reaction of aluminium ions with acetylacetone. It is a white, crystalline solid that is insoluble in water but soluble in organic solvents. This compound is widely used in various industrial and scientific applications due to its stability and reactivity.
Mechanism of Action
Target of Action
Aluminium acetylacetonate, also referred to as Al(acac)3, is a coordination complex with formula Al(C5H7O2)3 . This aluminium complex with three acetylacetone ligands is used in research on Al-containing materials . The molecule has D3 symmetry, being isomorphous with other octahedral tris(acetylacetonate)s .
Mode of Action
The dissociative ionization of the room temperature precursor molecule starts at a photon energy of 8.5 eV by the rupture of the bond to an acetylacetonate ligand leading to the formation of the Al(C5H7O2)2+ ion . In pyrolysis experiments, up to 49 species were detected and identified in the gas-phase, including reactive intermediates and isomeric/isobaric hydrocarbons, oxygenated species as well as aluminium containing molecules .
Biochemical Pathways
It has been studied in a microreactor by double imaging photoelectron photoion coincidence spectroscopy (i2pepico) between 325 and 1273 k . The major initial products formed at temperatures above 600 K are aluminium bis(diketo)acetylacetonate-H, Al(C5H7O2)C5H6O2, and acetylacetone (C5H8O2) .
Pharmacokinetics
It is known that the compound has a low solubility in water , which may impact its bioavailability.
Result of Action
The decomposition of this compound leads to the formation of various species, including reactive intermediates and isomeric/isobaric hydrocarbons, oxygenated species as well as aluminium containing molecules . These species can have various effects at the molecular and cellular level.
Action Environment
The action of this compound can be influenced by environmental factors such as temperature . For example, the decomposition of the compound and the resulting species formed can vary depending on the temperature
Biochemical Analysis
Biochemical Properties
Metal acetylacetonates are coordination complexes derived from the acetylacetonate anion and metal ions, usually transition metals . The ligand acetylacetonate is a β-diketone often abbreviated as “acac” . Acetylacetone is an organic compound that famously exists in two tautomeric forms which are rapidly inter-convertible . Typically both oxygen atoms bind to the metal to form a six-membered chelate ring . Metal enolates are widely used as building blocks in modern organic synthesis .
Cellular Effects
It has been found that while aluminum sulfate did not have an effect, aluminum acetylacetonate caused a dose-dependent and time-dependent decrease in the cell viability of the two cell lines tested .
Molecular Mechanism
It is known that metal acetylacetonates are used in a plethora of catalysed reactions . Several catalytic reactions have been highlighted to show where metal enolate complexes have played a significant role in recent years .
Temporal Effects in Laboratory Settings
The thermal decomposition of Aluminium acetylacetonate has been studied in a microreactor by double imaging photoelectron photoion coincidence spectroscopy (i2PEPIC) between 325 and 1273 K .
Metabolic Pathways
Metal acetylacetonates are coordination complexes derived from the acetylacetonate anion and metal ions, usually transition metals .
Transport and Distribution
It is known that metal acetylacetonates are coordination complexes derived from the acetylacetonate anion and metal ions, usually transition metals .
Preparation Methods
Synthetic Routes and Reaction Conditions: Aluminium acetylacetonate can be synthesized by reacting aluminium chloride with acetylacetone in the presence of a base. The reaction typically involves the following steps:
- Dissolve aluminium chloride in an organic solvent such as ethanol.
- Add acetylacetone to the solution.
- Introduce a base, such as sodium hydroxide, to facilitate the removal of a proton from acetylacetone, shifting the equilibrium towards the formation of the complex.
- The reaction mixture is then heated to promote the formation of this compound, which precipitates out of the solution .
Industrial Production Methods: In industrial settings, this compound is produced by a similar method but on a larger scale. The process involves:
- Adding isopropanol and acetone into a reactor and heating to 50-60°C.
- Slowly adding pure aluminium powder while controlling the temperature below 80°C.
- After the reaction is complete, acetylacetone is added, and the mixture is further heated to 100°C.
- The product is then cooled, centrifuged, filtered, and dried to obtain this compound .
Chemical Reactions Analysis
Types of Reactions: Aluminium acetylacetonate undergoes various chemical reactions, including:
Oxidation: It can be oxidized to form aluminium oxide.
Reduction: It can be reduced under specific conditions to yield aluminium metal.
Substitution: The acetylacetonate ligands can be substituted with other ligands in the presence of suitable reagents.
Common Reagents and Conditions:
Oxidation: Typically involves heating in the presence of oxygen or an oxidizing agent.
Reduction: Requires a reducing agent such as hydrogen gas or a metal hydride.
Substitution: Involves the use of other ligands and a suitable solvent to facilitate the exchange.
Major Products:
Oxidation: Aluminium oxide.
Reduction: Aluminium metal.
Substitution: Various substituted aluminium complexes depending on the ligands used.
Scientific Research Applications
Aluminium acetylacetonate has numerous applications in scientific research:
Biology: Employed in the synthesis of bioactive compounds and as a reagent in biochemical assays.
Medicine: Investigated for its potential use in drug delivery systems and as an adjuvant in vaccines.
Comparison with Similar Compounds
- Chromium acetylacetonate
- Iron acetylacetonate
- Copper acetylacetonate
Comparison:
- Stability: Aluminium acetylacetonate is more stable than some other metal acetylacetonates due to the strong chelation effect of the acetylacetonate ligands.
- Reactivity: It exhibits different reactivity patterns compared to other metal acetylacetonates, making it suitable for specific applications.
- Applications: While other metal acetylacetonates are also used as catalysts and in material science, this compound is particularly valued for its role in the production of aluminium oxide films and superhydrophobic coatings .
Properties
CAS No. |
13963-57-0 |
---|---|
Molecular Formula |
C15H21AlO6 |
Molecular Weight |
324.30 g/mol |
IUPAC Name |
aluminum;pentane-2,4-dione |
InChI |
InChI=1S/3C5H7O2.Al/c3*1-4(6)3-5(2)7;/h3*3H,1-2H3;/q3*-1;+3 |
InChI Key |
QWKOJPUROFIHOB-UHFFFAOYSA-N |
SMILES |
CC(=CC(=O)C)O[Al](OC(=CC(=O)C)C)OC(=CC(=O)C)C |
Isomeric SMILES |
C/C(=C/C(=O)C)/O[Al](O/C(=C\C(=O)C)/C)O/C(=C\C(=O)C)/C |
Canonical SMILES |
CC(=O)[CH-]C(=O)C.CC(=O)[CH-]C(=O)C.CC(=O)[CH-]C(=O)C.[Al+3] |
13963-57-0 | |
Pictograms |
Acute Toxic; Irritant |
Synonyms |
Al(ACAC)3 aluminum acetylacetonate aluminum(III) tris(acetylacetonate) aluminum(III)tris(acetylacetonate) tris(acetylacetonate) aluminum(III) |
Origin of Product |
United States |
Retrosynthesis Analysis
AI-Powered Synthesis Planning: Our tool employs the Template_relevance Pistachio, Template_relevance Bkms_metabolic, Template_relevance Pistachio_ringbreaker, Template_relevance Reaxys, Template_relevance Reaxys_biocatalysis model, leveraging a vast database of chemical reactions to predict feasible synthetic routes.
One-Step Synthesis Focus: Specifically designed for one-step synthesis, it provides concise and direct routes for your target compounds, streamlining the synthesis process.
Accurate Predictions: Utilizing the extensive PISTACHIO, BKMS_METABOLIC, PISTACHIO_RINGBREAKER, REAXYS, REAXYS_BIOCATALYSIS database, our tool offers high-accuracy predictions, reflecting the latest in chemical research and data.
Strategy Settings
Precursor scoring | Relevance Heuristic |
---|---|
Min. plausibility | 0.01 |
Model | Template_relevance |
Template Set | Pistachio/Bkms_metabolic/Pistachio_ringbreaker/Reaxys/Reaxys_biocatalysis |
Top-N result to add to graph | 6 |
Feasible Synthetic Routes
Disclaimer and Information on In-Vitro Research Products
Please be aware that all articles and product information presented on BenchChem are intended solely for informational purposes. The products available for purchase on BenchChem are specifically designed for in-vitro studies, which are conducted outside of living organisms. In-vitro studies, derived from the Latin term "in glass," involve experiments performed in controlled laboratory settings using cells or tissues. It is important to note that these products are not categorized as medicines or drugs, and they have not received approval from the FDA for the prevention, treatment, or cure of any medical condition, ailment, or disease. We must emphasize that any form of bodily introduction of these products into humans or animals is strictly prohibited by law. It is essential to adhere to these guidelines to ensure compliance with legal and ethical standards in research and experimentation.