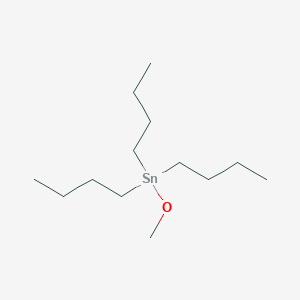
Tributyltin methoxide
Overview
Description
Tributyltin methoxide is an organotin compound with the molecular formula C₁₃H₃₀OSn and a molecular weight of 321.09 g/mol . It consists of a tin atom bonded to three butyl groups and one methoxide group. This compound is part of the broader class of tributyltin compounds, which have been widely studied for their various applications and effects.
Mechanism of Action
Tributyltin methoxide, also known as Tri-n-Butyltin Methoxide, is an organotin compound with the linear formula [CH3(CH2)3]3SnOCH3 . This compound has been recognized for its significant biological activity, particularly as an endocrine-disrupting chemical (EDC) .
Target of Action
The primary targets of this compound are the nuclear retinoid-X receptor (RXR) and peroxisome proliferator-activated receptor γ (PPARγ), and their heterodimers . These receptors play crucial roles in regulating gene expression, cellular differentiation, and metabolic processes .
Mode of Action
This compound interacts with RXR and PPARγ, leading to alterations in a range of reproductive, developmental, and metabolic pathways at the organism level . This interaction is considered the molecular initiating event (MIE) in the compound’s mechanism of action .
Biochemical Pathways
The interaction of this compound with RXR and PPARγ affects various biochemical pathways. These include pathways related to reproduction, development, and metabolism . The exact downstream effects can vary depending on the specific pathway and the organism .
Pharmacokinetics
The compound’s bioavailability is likely influenced by these properties .
Result of Action
The molecular and cellular effects of this compound’s action are significant. Its interaction with RXR and PPARγ can lead to changes in gene expression and cellular processes . This can result in a variety of physiological effects, including alterations in reproductive and metabolic functions .
Action Environment
Environmental factors can influence the action, efficacy, and stability of this compound. For instance, the compound is known to adhere to bed sediments in aquatic environments . Its half-life in marine water is about one to two weeks, but when it accumulates in sediments, its half-life extends to about two years . Moreover, TBT can remain in sediments and be released back into the aquatic environment for up to 30 years .
Biochemical Analysis
Biochemical Properties
Tributyltin Methoxide interacts with various enzymes, proteins, and other biomolecules. Its primary endocrine mechanism of action involves interactions with the nuclear retinoid-X receptor (RXR) and peroxisome proliferator-activated receptor γ (PPARγ), and their heterodimers . This interaction alters a range of reproductive, developmental, and metabolic pathways at the organism level .
Cellular Effects
This compound has been found to have significant effects on various types of cells and cellular processes. It influences cell function, including impacts on cell signaling pathways, gene expression, and cellular metabolism . For instance, it has been shown to increase glucose-stimulated insulin secretion and intracellular Ca 2+ in β-cells .
Molecular Mechanism
The molecular mechanism of action of this compound involves its binding interactions with biomolecules, enzyme inhibition or activation, and changes in gene expression . It exerts its effects at the molecular level primarily through interactions with the nuclear retinoid-X receptor (RXR), peroxisome proliferator-activated receptor γ (PPARγ), and their heterodimers .
Temporal Effects in Laboratory Settings
In laboratory settings, the effects of this compound change over time. It has been shown to induce insulin dysregulation and disturb glucose homeostasis, which may be mediated through the estrogen receptor-regulated and/or oxidative stress-related signaling pathways .
Dosage Effects in Animal Models
The effects of this compound vary with different dosages in animal models. For instance, small doses of this compound (100 and 500 ng/kg/bw/day for 15 days) modified the vascular reactivity in aorta, mesenteric and coronary arteries in an experimental rodent model .
Metabolic Pathways
This compound is involved in various metabolic pathways. It can be metabolized sequentially by cytochrome P450 (CYP450) enzymes to dibutyl- and monobutyltin .
Preparation Methods
Tributyltin methoxide can be synthesized through the reaction of tributyltin chloride with sodium methoxide. The reaction typically occurs in an inert atmosphere to prevent oxidation and is carried out in a suitable solvent such as tetrahydrofuran. The reaction conditions include maintaining a low temperature to control the reaction rate and prevent side reactions .
Reaction:
(C4H9)3SnCl+NaOCH3→(C4H9)3SnOCH3+NaCl
In industrial settings, the production of this compound follows similar principles but on a larger scale, with careful control of reaction parameters to ensure high yield and purity.
Chemical Reactions Analysis
Tributyltin methoxide undergoes various types of chemical reactions, including:
Substitution Reactions: It can react with halides, forming new organotin compounds.
Addition Reactions: It can add to multiple bonds in compounds such as isothiocyanates, trichloroacetonitrile, and carbon disulfide.
Oxidation and Reduction Reactions: this compound can participate in redox reactions, although specific conditions and reagents vary.
Common Reagents and Conditions:
Halides: Used in substitution reactions.
Multiple Bond Compounds: Used in addition reactions.
Oxidizing and Reducing Agents: Used in redox reactions.
Major Products:
- New organotin compounds with different functional groups.
- Addition products with modified chemical structures.
Scientific Research Applications
Tributyltin methoxide has several applications in scientific research:
Chemistry: Used as a reagent in organic synthesis for forming carbon-tin bonds.
Biology: Studied for its effects on biological systems, particularly its endocrine-disrupting properties.
Medicine: Investigated for potential therapeutic applications, although its toxicity limits its use.
Comparison with Similar Compounds
- Tributyltin chloride
- Tributyltin oxide
- Tributyltin hydride
Uniqueness:
- The methoxide group in tributyltin methoxide provides distinct reactivity and potential applications in organic synthesis and industrial processes.
Properties
IUPAC Name |
tributyl(methoxy)stannane | |
---|---|---|
Source | PubChem | |
URL | https://pubchem.ncbi.nlm.nih.gov | |
Description | Data deposited in or computed by PubChem | |
InChI |
InChI=1S/3C4H9.CH3O.Sn/c3*1-3-4-2;1-2;/h3*1,3-4H2,2H3;1H3;/q;;;-1;+1 | |
Source | PubChem | |
URL | https://pubchem.ncbi.nlm.nih.gov | |
Description | Data deposited in or computed by PubChem | |
InChI Key |
KJGLZJQPMKQFIK-UHFFFAOYSA-N | |
Source | PubChem | |
URL | https://pubchem.ncbi.nlm.nih.gov | |
Description | Data deposited in or computed by PubChem | |
Canonical SMILES |
CCCC[Sn](CCCC)(CCCC)OC | |
Source | PubChem | |
URL | https://pubchem.ncbi.nlm.nih.gov | |
Description | Data deposited in or computed by PubChem | |
Molecular Formula |
C13H30OSn | |
Source | PubChem | |
URL | https://pubchem.ncbi.nlm.nih.gov | |
Description | Data deposited in or computed by PubChem | |
DSSTOX Substance ID |
DTXSID3074440 | |
Record name | Stannane, tributylmethoxy- | |
Source | EPA DSSTox | |
URL | https://comptox.epa.gov/dashboard/DTXSID3074440 | |
Description | DSSTox provides a high quality public chemistry resource for supporting improved predictive toxicology. | |
Molecular Weight |
321.09 g/mol | |
Source | PubChem | |
URL | https://pubchem.ncbi.nlm.nih.gov | |
Description | Data deposited in or computed by PubChem | |
CAS No. |
1067-52-3 | |
Record name | Tributylmethoxystannane | |
Source | CAS Common Chemistry | |
URL | https://commonchemistry.cas.org/detail?cas_rn=1067-52-3 | |
Description | CAS Common Chemistry is an open community resource for accessing chemical information. Nearly 500,000 chemical substances from CAS REGISTRY cover areas of community interest, including common and frequently regulated chemicals, and those relevant to high school and undergraduate chemistry classes. This chemical information, curated by our expert scientists, is provided in alignment with our mission as a division of the American Chemical Society. | |
Explanation | The data from CAS Common Chemistry is provided under a CC-BY-NC 4.0 license, unless otherwise stated. | |
Record name | Tributyltin methoxide | |
Source | ChemIDplus | |
URL | https://pubchem.ncbi.nlm.nih.gov/substance/?source=chemidplus&sourceid=0001067523 | |
Description | ChemIDplus is a free, web search system that provides access to the structure and nomenclature authority files used for the identification of chemical substances cited in National Library of Medicine (NLM) databases, including the TOXNET system. | |
Record name | Tributyltin methoxide | |
Source | DTP/NCI | |
URL | https://dtp.cancer.gov/dtpstandard/servlet/dwindex?searchtype=NSC&outputformat=html&searchlist=202857 | |
Description | The NCI Development Therapeutics Program (DTP) provides services and resources to the academic and private-sector research communities worldwide to facilitate the discovery and development of new cancer therapeutic agents. | |
Explanation | Unless otherwise indicated, all text within NCI products is free of copyright and may be reused without our permission. Credit the National Cancer Institute as the source. | |
Record name | Stannane, tributylmethoxy- | |
Source | EPA DSSTox | |
URL | https://comptox.epa.gov/dashboard/DTXSID3074440 | |
Description | DSSTox provides a high quality public chemistry resource for supporting improved predictive toxicology. | |
Record name | Tri-n-butyltin methanolate | |
Source | European Chemicals Agency (ECHA) | |
URL | https://echa.europa.eu/substance-information/-/substanceinfo/100.012.667 | |
Description | The European Chemicals Agency (ECHA) is an agency of the European Union which is the driving force among regulatory authorities in implementing the EU's groundbreaking chemicals legislation for the benefit of human health and the environment as well as for innovation and competitiveness. | |
Explanation | Use of the information, documents and data from the ECHA website is subject to the terms and conditions of this Legal Notice, and subject to other binding limitations provided for under applicable law, the information, documents and data made available on the ECHA website may be reproduced, distributed and/or used, totally or in part, for non-commercial purposes provided that ECHA is acknowledged as the source: "Source: European Chemicals Agency, http://echa.europa.eu/". Such acknowledgement must be included in each copy of the material. ECHA permits and encourages organisations and individuals to create links to the ECHA website under the following cumulative conditions: Links can only be made to webpages that provide a link to the Legal Notice page. | |
Record name | TRIBUTYLTIN METHOXIDE | |
Source | FDA Global Substance Registration System (GSRS) | |
URL | https://gsrs.ncats.nih.gov/ginas/app/beta/substances/9CFF2AFN2V | |
Description | The FDA Global Substance Registration System (GSRS) enables the efficient and accurate exchange of information on what substances are in regulated products. Instead of relying on names, which vary across regulatory domains, countries, and regions, the GSRS knowledge base makes it possible for substances to be defined by standardized, scientific descriptions. | |
Explanation | Unless otherwise noted, the contents of the FDA website (www.fda.gov), both text and graphics, are not copyrighted. They are in the public domain and may be republished, reprinted and otherwise used freely by anyone without the need to obtain permission from FDA. Credit to the U.S. Food and Drug Administration as the source is appreciated but not required. | |
Retrosynthesis Analysis
AI-Powered Synthesis Planning: Our tool employs the Template_relevance Pistachio, Template_relevance Bkms_metabolic, Template_relevance Pistachio_ringbreaker, Template_relevance Reaxys, Template_relevance Reaxys_biocatalysis model, leveraging a vast database of chemical reactions to predict feasible synthetic routes.
One-Step Synthesis Focus: Specifically designed for one-step synthesis, it provides concise and direct routes for your target compounds, streamlining the synthesis process.
Accurate Predictions: Utilizing the extensive PISTACHIO, BKMS_METABOLIC, PISTACHIO_RINGBREAKER, REAXYS, REAXYS_BIOCATALYSIS database, our tool offers high-accuracy predictions, reflecting the latest in chemical research and data.
Strategy Settings
Precursor scoring | Relevance Heuristic |
---|---|
Min. plausibility | 0.01 |
Model | Template_relevance |
Template Set | Pistachio/Bkms_metabolic/Pistachio_ringbreaker/Reaxys/Reaxys_biocatalysis |
Top-N result to add to graph | 6 |
Feasible Synthetic Routes
Q1: What is the molecular formula, weight, and key spectroscopic data for TBTMO?
A1: TBTMO has the molecular formula C13H30OSn and a molecular weight of 321.14 g/mol. Key spectroscopic data includes a 1H NMR signal at 3.54 ppm for the methoxy group (OMe) and a 119Sn NMR signal at 83 ppm [].
Q2: What is the significance of the Sn-O bond in TBTMO?
A2: The Sn-O bond in TBTMO plays a crucial role in its reactivity. This bond readily adds across carbonyl groups, enabling reactions with aldehydes, ketones, and even chloral [, ].
Q3: How does TBTMO act as a catalyst in ring-opening polymerization?
A3: TBTMO effectively catalyzes the ring-opening polymerization of lactones, such as β-butyrolactone and ε-caprolactone [, , ]. The mechanism involves the Sn-O bond coordinating to the carbonyl group of the lactone, facilitating ring-opening and subsequent chain propagation.
Q4: Can TBTMO catalyze syndiospecific polymerization?
A4: Yes, TBTMO exhibits a unique ability to catalyze the syndiospecific polymerization of β-butyrolactone, leading to predominantly syndiotactic poly(β-hydroxybutyrate) [, ]. This selectivity is attributed to chain-end stereocontrol during the polymerization process.
Q5: Are there other catalytic applications of TBTMO?
A5: TBTMO acts as a catalyst in various other reactions, including:
- Transesterification: It facilitates the transesterification of poly(L-lactide) with poly(ε-caprolactone), forming copolymers with slightly blocky sequences [].
- Direct synthesis of alkynylstannanes: TBTMO reacts with terminal alkynes in the presence of ZnBr2 as a catalyst, providing a convenient route to alkynylstannanes [, ].
Q6: How can TBTMO be used to synthesize vinyltin compounds?
A6: While direct hydrostannation of but-3-ynoic acid with tributyltin hydride yields the tributyltin carboxylate, using two equivalents of tributyltin hydride in the presence of TBTMO leads to the desired protected vinyltin compound [].
Q7: Can TBTMO be used for the protection of carboxylic acids?
A7: Yes, TBTMO offers a convenient method for temporarily protecting carboxylic acids as tributyltin esters. Deprotection can be achieved using potassium fluoride followed by a mildly acidic solution [].
Q8: What are the applications of TBTMO in palladium-catalyzed coupling reactions?
A8: TBTMO plays a crucial role in several palladium-catalyzed reactions, such as:
- α-Phenylation of ketones: TBTMO generates tin enolates in situ from enol acetates, which then undergo palladium-catalyzed coupling with bromobenzene to yield α-phenyl ketones [].
- Synthesis of aryl ketones: TBTMO facilitates the coupling of aryl bromides with vinylic acetates in the presence of a palladium catalyst, resulting in the unexpected formation of aryl ketones [, ].
Q9: What is known about the toxicity of TBTMO?
A9: TBTMO exhibits acute toxicity, with an LD50 of 98 mg/kg in mice []. Studies have shown that it can induce sperm abnormalities in a dose-dependent manner and may have genotoxic potential [].
Disclaimer and Information on In-Vitro Research Products
Please be aware that all articles and product information presented on BenchChem are intended solely for informational purposes. The products available for purchase on BenchChem are specifically designed for in-vitro studies, which are conducted outside of living organisms. In-vitro studies, derived from the Latin term "in glass," involve experiments performed in controlled laboratory settings using cells or tissues. It is important to note that these products are not categorized as medicines or drugs, and they have not received approval from the FDA for the prevention, treatment, or cure of any medical condition, ailment, or disease. We must emphasize that any form of bodily introduction of these products into humans or animals is strictly prohibited by law. It is essential to adhere to these guidelines to ensure compliance with legal and ethical standards in research and experimentation.