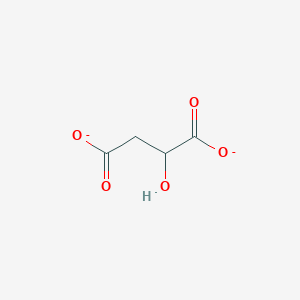
Malate
Overview
Description
Malate, also known as 2-hydroxybutanedioic acid, is a dicarboxylic acid that plays a crucial role in the citric acid cycle, which is a key metabolic pathway in all aerobic organisms. It is naturally found in many fruits, especially apples, and contributes to their sour taste. This compound exists in two stereoisomeric forms: L-malate and D-malate, with L-malate being the naturally occurring form.
Preparation Methods
Synthetic Routes and Reaction Conditions
Biocatalysis: One of the primary methods for producing L-malate is through the enzymatic transformation of fumarate to L-malate.
Microbial Fermentation: Another method involves the use of genetically engineered microorganisms to convert substrates like glucose into L-malate.
Chemical Synthesis: L-malate can also be synthesized chemically by the hydration of maleic acid or fumaric acid.
Industrial Production Methods
Industrial production of L-malate often employs microbial fermentation due to its cost-effectiveness and sustainability. The process involves the use of industrially relevant strains of microorganisms that are engineered to enhance malate production. The fermentation is carried out in large bioreactors with continuous monitoring and optimization of conditions to maximize yield .
Chemical Reactions Analysis
Types of Reactions
Reduction: This compound can be reduced to succinate in the glyoxylate cycle, a variation of the citric acid cycle.
Common Reagents and Conditions
Oxidation: Requires this compound dehydrogenase and NAD+ as a cofactor.
Reduction: Involves enzymes like this compound synthase and specific cofactors.
Substitution: Typically requires acidic or basic conditions and specific catalysts depending on the desired product
Major Products
Oxaloacetate: Formed from the oxidation of this compound.
Succinate: Produced from the reduction of this compound.
This compound Esters: Result from substitution reactions involving this compound
Scientific Research Applications
Malate has a wide range of applications in scientific research:
Chemistry: Used as a chiral building block in the synthesis of various organic compounds.
Biology: Plays a critical role in cellular respiration and energy production.
Medicine: this compound derivatives, such as citrulline this compound, are used to enhance exercise performance and reduce muscle fatigue.
Industry: Used as an acidulant and flavor enhancer in the food and beverage industry.
Mechanism of Action
Malate exerts its effects primarily through its role in the citric acid cycle. It is converted to oxaloacetate by this compound dehydrogenase, which is a key step in the cycle that generates energy in the form of adenosine triphosphate (ATP). This compound also acts as a carbon source for the synthesis of other important biomolecules and plays a role in the regulation of cellular pH .
Comparison with Similar Compounds
Similar Compounds
Succinic Acid: Another dicarboxylic acid involved in the citric acid cycle.
Fumaric Acid: An intermediate in the citric acid cycle that is converted to malate.
Tartaric Acid: A dicarboxylic acid similar in structure to this compound but with different functional properties
Uniqueness of this compound
This compound is unique due to its dual role in both the citric acid cycle and the glyoxylate cycle. It serves as a key intermediate in energy production and metabolic regulation. Additionally, its ability to undergo various chemical reactions makes it a versatile compound in both biological and industrial applications .
Properties
IUPAC Name |
2-hydroxybutanedioate | |
---|---|---|
Source | PubChem | |
URL | https://pubchem.ncbi.nlm.nih.gov | |
Description | Data deposited in or computed by PubChem | |
InChI |
InChI=1S/C4H6O5/c5-2(4(8)9)1-3(6)7/h2,5H,1H2,(H,6,7)(H,8,9)/p-2 | |
Source | PubChem | |
URL | https://pubchem.ncbi.nlm.nih.gov | |
Description | Data deposited in or computed by PubChem | |
InChI Key |
BJEPYKJPYRNKOW-UHFFFAOYSA-L | |
Source | PubChem | |
URL | https://pubchem.ncbi.nlm.nih.gov | |
Description | Data deposited in or computed by PubChem | |
Canonical SMILES |
C(C(C(=O)[O-])O)C(=O)[O-] | |
Source | PubChem | |
URL | https://pubchem.ncbi.nlm.nih.gov | |
Description | Data deposited in or computed by PubChem | |
Molecular Formula |
C4H4O5-2 | |
Source | PubChem | |
URL | https://pubchem.ncbi.nlm.nih.gov | |
Description | Data deposited in or computed by PubChem | |
DSSTOX Substance ID |
DTXSID00933521 | |
Record name | 2-Hydroxybutanedioate | |
Source | EPA DSSTox | |
URL | https://comptox.epa.gov/dashboard/DTXSID00933521 | |
Description | DSSTox provides a high quality public chemistry resource for supporting improved predictive toxicology. | |
Molecular Weight |
132.07 g/mol | |
Source | PubChem | |
URL | https://pubchem.ncbi.nlm.nih.gov | |
Description | Data deposited in or computed by PubChem | |
CAS No. |
149-61-1 | |
Record name | DL-Malate | |
Source | CAS Common Chemistry | |
URL | https://commonchemistry.cas.org/detail?cas_rn=149-61-1 | |
Description | CAS Common Chemistry is an open community resource for accessing chemical information. Nearly 500,000 chemical substances from CAS REGISTRY cover areas of community interest, including common and frequently regulated chemicals, and those relevant to high school and undergraduate chemistry classes. This chemical information, curated by our expert scientists, is provided in alignment with our mission as a division of the American Chemical Society. | |
Explanation | The data from CAS Common Chemistry is provided under a CC-BY-NC 4.0 license, unless otherwise stated. | |
Record name | Butanedioic acid, hydroxy-, ion(2)- | |
Source | ChemIDplus | |
URL | https://pubchem.ncbi.nlm.nih.gov/substance/?source=chemidplus&sourceid=0000149611 | |
Description | ChemIDplus is a free, web search system that provides access to the structure and nomenclature authority files used for the identification of chemical substances cited in National Library of Medicine (NLM) databases, including the TOXNET system. | |
Record name | 2-Hydroxybutanedioate | |
Source | EPA DSSTox | |
URL | https://comptox.epa.gov/dashboard/DTXSID00933521 | |
Description | DSSTox provides a high quality public chemistry resource for supporting improved predictive toxicology. | |
Synthesis routes and methods I
Procedure details
Synthesis routes and methods II
Procedure details
Synthesis routes and methods III
Procedure details
Synthesis routes and methods IV
Procedure details
Synthesis routes and methods V
Procedure details
Retrosynthesis Analysis
AI-Powered Synthesis Planning: Our tool employs the Template_relevance Pistachio, Template_relevance Bkms_metabolic, Template_relevance Pistachio_ringbreaker, Template_relevance Reaxys, Template_relevance Reaxys_biocatalysis model, leveraging a vast database of chemical reactions to predict feasible synthetic routes.
One-Step Synthesis Focus: Specifically designed for one-step synthesis, it provides concise and direct routes for your target compounds, streamlining the synthesis process.
Accurate Predictions: Utilizing the extensive PISTACHIO, BKMS_METABOLIC, PISTACHIO_RINGBREAKER, REAXYS, REAXYS_BIOCATALYSIS database, our tool offers high-accuracy predictions, reflecting the latest in chemical research and data.
Strategy Settings
Precursor scoring | Relevance Heuristic |
---|---|
Min. plausibility | 0.01 |
Model | Template_relevance |
Template Set | Pistachio/Bkms_metabolic/Pistachio_ringbreaker/Reaxys/Reaxys_biocatalysis |
Top-N result to add to graph | 6 |
Feasible Synthetic Routes
Disclaimer and Information on In-Vitro Research Products
Please be aware that all articles and product information presented on BenchChem are intended solely for informational purposes. The products available for purchase on BenchChem are specifically designed for in-vitro studies, which are conducted outside of living organisms. In-vitro studies, derived from the Latin term "in glass," involve experiments performed in controlled laboratory settings using cells or tissues. It is important to note that these products are not categorized as medicines or drugs, and they have not received approval from the FDA for the prevention, treatment, or cure of any medical condition, ailment, or disease. We must emphasize that any form of bodily introduction of these products into humans or animals is strictly prohibited by law. It is essential to adhere to these guidelines to ensure compliance with legal and ethical standards in research and experimentation.