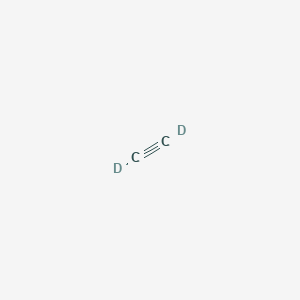
Acetylene-d2
Overview
Description
Acetylene-d2, also known as deuterated acetylene, is a deuterium-labeled variant of acetylene. It has the molecular formula C2D2, where the hydrogen atoms in acetylene (C2H2) are replaced by deuterium atoms. Deuterium is an isotope of hydrogen with an additional neutron, making it twice as heavy as hydrogen. This compound is used extensively in scientific research due to its unique properties, which include altered vibrational frequencies and reaction kinetics compared to its non-deuterated counterpart.
Preparation Methods
Synthetic Routes and Reaction Conditions: Acetylene-d2 can be synthesized through the reaction of calcium carbide with deuterium oxide (heavy water). The reaction proceeds as follows: [ \text{CaC}_2 + 2\text{D}_2\text{O} \rightarrow \text{C}_2\text{D}_2 + \text{Ca(OD)}_2 ] This method involves the careful handling of calcium carbide and deuterium oxide under controlled conditions to ensure the purity and yield of this compound.
Industrial Production Methods: In an industrial setting, this compound can be produced using a similar approach but on a larger scale. The process involves the use of specialized reactors and purification systems to handle the reactants and products efficiently. The production of this compound requires stringent quality control measures to ensure the isotopic purity of the final product.
Chemical Reactions Analysis
Types of Reactions: Acetylene-d2 undergoes various chemical reactions, including:
Hydrogenation (Deuteration): this compound can be hydrogenated to form deuterated ethylene (C2D4) using catalysts such as palladium or platinum.
Halogenation: this compound reacts with halogens like bromine to form deuterated dihaloalkenes.
Addition Reactions: this compound can participate in addition reactions with compounds like hydrogen bromide to form deuterated bromoalkenes.
Common Reagents and Conditions:
Hydrogenation: Catalysts like palladium or platinum are used under mild conditions.
Halogenation: Halogens such as bromine or chlorine are used, often in the presence of a solvent like carbon tetrachloride.
Addition Reactions: Hydrogen halides are used, typically under controlled temperature and pressure conditions.
Major Products:
Deuterated Ethylene (C2D4): Formed from hydrogenation.
Deuterated Dihaloalkenes: Formed from halogenation.
Deuterated Bromoalkenes: Formed from addition reactions with hydrogen bromide.
Scientific Research Applications
Acetylene-d2 is used in various scientific research applications, including:
Chemistry: It is used as a tracer in reaction mechanisms to study the kinetics and pathways of chemical reactions.
Biology: this compound is used in metabolic studies to trace the incorporation of deuterium into biological molecules.
Medicine: It is used in the development of deuterated drugs, which can have altered pharmacokinetics and improved stability.
Industry: this compound is used in the production of deuterated polymers and other materials with unique properties.
Mechanism of Action
The mechanism by which acetylene-d2 exerts its effects is primarily through its participation in chemical reactions. The presence of deuterium atoms alters the vibrational frequencies and bond strengths compared to hydrogen, leading to differences in reaction kinetics and pathways. These differences can be exploited to study reaction mechanisms and develop new materials with enhanced properties.
Comparison with Similar Compounds
Acetylene (C2H2): The non-deuterated counterpart of acetylene-d2.
Deuterated Ethylene (C2D4): A product of the hydrogenation of this compound.
Deuterated Bromoalkenes: Products of the addition of hydrogen bromide to this compound.
Comparison:
Acetylene (C2H2): this compound has a higher molecular weight and different vibrational frequencies due to the presence of deuterium.
Deuterated Ethylene (C2D4): Similar in structure but differs in the number of deuterium atoms.
Deuterated Bromoalkenes: These compounds have additional functional groups compared to this compound, leading to different chemical properties and reactivity.
This compound stands out due to its unique isotopic composition, making it a valuable tool in scientific research and industrial applications. Its ability to provide insights into reaction mechanisms and develop new materials highlights its significance in various fields.
Properties
IUPAC Name |
1,2-dideuterioethyne | |
---|---|---|
Source | PubChem | |
URL | https://pubchem.ncbi.nlm.nih.gov | |
Description | Data deposited in or computed by PubChem | |
InChI |
InChI=1S/C2H2/c1-2/h1-2H/i1D,2D | |
Source | PubChem | |
URL | https://pubchem.ncbi.nlm.nih.gov | |
Description | Data deposited in or computed by PubChem | |
InChI Key |
HSFWRNGVRCDJHI-QDNHWIQGSA-N | |
Source | PubChem | |
URL | https://pubchem.ncbi.nlm.nih.gov | |
Description | Data deposited in or computed by PubChem | |
Canonical SMILES |
C#C | |
Source | PubChem | |
URL | https://pubchem.ncbi.nlm.nih.gov | |
Description | Data deposited in or computed by PubChem | |
Isomeric SMILES |
[2H]C#C[2H] | |
Source | PubChem | |
URL | https://pubchem.ncbi.nlm.nih.gov | |
Description | Data deposited in or computed by PubChem | |
Molecular Formula |
C2H2 | |
Source | PubChem | |
URL | https://pubchem.ncbi.nlm.nih.gov | |
Description | Data deposited in or computed by PubChem | |
Related CAS |
28265-43-2 | |
Record name | Ethyne-1,2-d2, homopolymer | |
Source | CAS Common Chemistry | |
URL | https://commonchemistry.cas.org/detail?cas_rn=28265-43-2 | |
Description | CAS Common Chemistry is an open community resource for accessing chemical information. Nearly 500,000 chemical substances from CAS REGISTRY cover areas of community interest, including common and frequently regulated chemicals, and those relevant to high school and undergraduate chemistry classes. This chemical information, curated by our expert scientists, is provided in alignment with our mission as a division of the American Chemical Society. | |
Explanation | The data from CAS Common Chemistry is provided under a CC-BY-NC 4.0 license, unless otherwise stated. | |
DSSTOX Substance ID |
DTXSID00147868 | |
Record name | Acetylene-d2 | |
Source | EPA DSSTox | |
URL | https://comptox.epa.gov/dashboard/DTXSID00147868 | |
Description | DSSTox provides a high quality public chemistry resource for supporting improved predictive toxicology. | |
Molecular Weight |
28.05 g/mol | |
Source | PubChem | |
URL | https://pubchem.ncbi.nlm.nih.gov | |
Description | Data deposited in or computed by PubChem | |
Physical Description |
Gas; [Sigma-Aldrich MSDS] | |
Record name | Acetylene-d2 | |
Source | Haz-Map, Information on Hazardous Chemicals and Occupational Diseases | |
URL | https://haz-map.com/Agents/13648 | |
Description | Haz-Map® is an occupational health database designed for health and safety professionals and for consumers seeking information about the adverse effects of workplace exposures to chemical and biological agents. | |
Explanation | Copyright (c) 2022 Haz-Map(R). All rights reserved. Unless otherwise indicated, all materials from Haz-Map are copyrighted by Haz-Map(R). No part of these materials, either text or image may be used for any purpose other than for personal use. Therefore, reproduction, modification, storage in a retrieval system or retransmission, in any form or by any means, electronic, mechanical or otherwise, for reasons other than personal use, is strictly prohibited without prior written permission. | |
CAS No. |
1070-74-2 | |
Record name | Ethyne-1,2-d2 | |
Source | CAS Common Chemistry | |
URL | https://commonchemistry.cas.org/detail?cas_rn=1070-74-2 | |
Description | CAS Common Chemistry is an open community resource for accessing chemical information. Nearly 500,000 chemical substances from CAS REGISTRY cover areas of community interest, including common and frequently regulated chemicals, and those relevant to high school and undergraduate chemistry classes. This chemical information, curated by our expert scientists, is provided in alignment with our mission as a division of the American Chemical Society. | |
Explanation | The data from CAS Common Chemistry is provided under a CC-BY-NC 4.0 license, unless otherwise stated. | |
Record name | Acetylene-d2 | |
Source | ChemIDplus | |
URL | https://pubchem.ncbi.nlm.nih.gov/substance/?source=chemidplus&sourceid=0001070742 | |
Description | ChemIDplus is a free, web search system that provides access to the structure and nomenclature authority files used for the identification of chemical substances cited in National Library of Medicine (NLM) databases, including the TOXNET system. | |
Record name | Acetylene-d2 | |
Source | EPA DSSTox | |
URL | https://comptox.epa.gov/dashboard/DTXSID00147868 | |
Description | DSSTox provides a high quality public chemistry resource for supporting improved predictive toxicology. | |
Record name | 1070-74-2 | |
Source | European Chemicals Agency (ECHA) | |
URL | https://echa.europa.eu/information-on-chemicals | |
Description | The European Chemicals Agency (ECHA) is an agency of the European Union which is the driving force among regulatory authorities in implementing the EU's groundbreaking chemicals legislation for the benefit of human health and the environment as well as for innovation and competitiveness. | |
Explanation | Use of the information, documents and data from the ECHA website is subject to the terms and conditions of this Legal Notice, and subject to other binding limitations provided for under applicable law, the information, documents and data made available on the ECHA website may be reproduced, distributed and/or used, totally or in part, for non-commercial purposes provided that ECHA is acknowledged as the source: "Source: European Chemicals Agency, http://echa.europa.eu/". Such acknowledgement must be included in each copy of the material. ECHA permits and encourages organisations and individuals to create links to the ECHA website under the following cumulative conditions: Links can only be made to webpages that provide a link to the Legal Notice page. | |
Retrosynthesis Analysis
AI-Powered Synthesis Planning: Our tool employs the Template_relevance Pistachio, Template_relevance Bkms_metabolic, Template_relevance Pistachio_ringbreaker, Template_relevance Reaxys, Template_relevance Reaxys_biocatalysis model, leveraging a vast database of chemical reactions to predict feasible synthetic routes.
One-Step Synthesis Focus: Specifically designed for one-step synthesis, it provides concise and direct routes for your target compounds, streamlining the synthesis process.
Accurate Predictions: Utilizing the extensive PISTACHIO, BKMS_METABOLIC, PISTACHIO_RINGBREAKER, REAXYS, REAXYS_BIOCATALYSIS database, our tool offers high-accuracy predictions, reflecting the latest in chemical research and data.
Strategy Settings
Precursor scoring | Relevance Heuristic |
---|---|
Min. plausibility | 0.01 |
Model | Template_relevance |
Template Set | Pistachio/Bkms_metabolic/Pistachio_ringbreaker/Reaxys/Reaxys_biocatalysis |
Top-N result to add to graph | 6 |
Feasible Synthetic Routes
Disclaimer and Information on In-Vitro Research Products
Please be aware that all articles and product information presented on BenchChem are intended solely for informational purposes. The products available for purchase on BenchChem are specifically designed for in-vitro studies, which are conducted outside of living organisms. In-vitro studies, derived from the Latin term "in glass," involve experiments performed in controlled laboratory settings using cells or tissues. It is important to note that these products are not categorized as medicines or drugs, and they have not received approval from the FDA for the prevention, treatment, or cure of any medical condition, ailment, or disease. We must emphasize that any form of bodily introduction of these products into humans or animals is strictly prohibited by law. It is essential to adhere to these guidelines to ensure compliance with legal and ethical standards in research and experimentation.