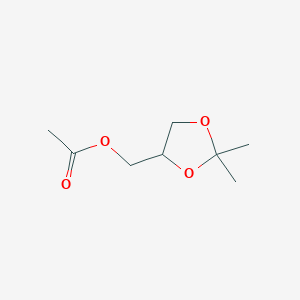
(2,2-Dimethyl-1,3-dioxolan-4-yl)methyl acetate
Overview
Description
(2,2-Dimethyl-1,3-dioxolan-4-yl)methyl acetate is an organic compound with the molecular formula C8H14O4. It is a derivative of dioxolane and is commonly used in various chemical reactions and industrial applications due to its unique structural properties.
Preparation Methods
Synthetic Routes and Reaction Conditions
The synthesis of (2,2-Dimethyl-1,3-dioxolan-4-yl)methyl acetate typically involves the reaction of 2,2-dimethyl-1,3-dioxolane-4-methanol with acetic anhydride or acetyl chloride. The reaction is usually carried out in the presence of a base such as pyridine or triethylamine to neutralize the hydrogen chloride formed during the reaction. The reaction conditions often include a temperature range of 0-25°C and a reaction time of 1-2 hours .
Industrial Production Methods
In industrial settings, the production of this compound is scaled up using continuous flow reactors to ensure consistent product quality and yield. The use of catalysts such as sulfuric acid or p-toluenesulfonic acid can enhance the reaction rate and efficiency .
Chemical Reactions Analysis
Types of Reactions
(2,2-Dimethyl-1,3-dioxolan-4-yl)methyl acetate undergoes various chemical reactions, including:
Hydrolysis: In the presence of water and an acid or base catalyst, it can hydrolyze to form 2,2-dimethyl-1,3-dioxolane-4-methanol and acetic acid.
Oxidation: It can be oxidized using reagents such as potassium permanganate or chromium trioxide to form corresponding carboxylic acids.
Substitution: It can undergo nucleophilic substitution reactions where the acetate group is replaced by other nucleophiles such as amines or alcohols.
Common Reagents and Conditions
Hydrolysis: Acidic or basic conditions with water.
Oxidation: Potassium permanganate or chromium trioxide in an acidic medium.
Substitution: Nucleophiles like amines or alcohols in the presence of a base.
Major Products Formed
Hydrolysis: 2,2-Dimethyl-1,3-dioxolane-4-methanol and acetic acid.
Oxidation: Corresponding carboxylic acids.
Substitution: Various substituted dioxolane derivatives.
Scientific Research Applications
(2,2-Dimethyl-1,3-dioxolan-4-yl)methyl acetate is used in several scientific research applications:
Chemistry: As a reagent in organic synthesis for the preparation of various dioxolane derivatives.
Biology: In the study of enzyme-catalyzed reactions involving ester hydrolysis.
Medicine: As an intermediate in the synthesis of pharmaceutical compounds.
Industry: In the production of polymers and resins due to its ability to undergo polymerization reactions.
Mechanism of Action
The mechanism of action of (2,2-Dimethyl-1,3-dioxolan-4-yl)methyl acetate involves its ability to act as an electrophile in various chemical reactions. It can form covalent bonds with nucleophiles, leading to the formation of new chemical compounds. The molecular targets and pathways involved include ester hydrolysis and nucleophilic substitution reactions .
Comparison with Similar Compounds
Similar Compounds
- Methyl 2-(2,2-dimethyl-1,3-dioxolan-4-yl)-2-(ethoxycarbonyloxy)acetate
- (2,2-Dimethyl-1,3-dioxolan-4-yl)methyl butyrate
- (2,2-Dimethyl-1,3-dioxolan-4-yl)methyl 3-oxobutanoate
Uniqueness
(2,2-Dimethyl-1,3-dioxolan-4-yl)methyl acetate is unique due to its specific structural configuration, which imparts distinct reactivity and stability compared to other similar compounds. Its ability to undergo a wide range of chemical reactions makes it a versatile reagent in both research and industrial applications .
Biological Activity
(2,2-Dimethyl-1,3-dioxolan-4-yl)methyl acetate, commonly referred to as solketal acetate, is an acetal compound derived from glycerol. Its biological activity has garnered attention due to its potential applications in biodiesel production and as a fuel additive. This article explores its synthesis, biological properties, and applications based on diverse research findings.
Solketal acetate can be synthesized through the acetalization of glycerol with acetone in the presence of acid catalysts. The reaction typically yields a mixture of solketal acetate and other acetal forms. The efficiency of this synthesis can be affected by various factors, such as the choice of catalyst and reaction conditions.
Reaction Mechanism
The acetalization process involves the formation of a stable dioxolane ring structure, which enhances the compound's stability and reactivity. The following table summarizes key parameters influencing the synthesis:
Parameter | Optimal Conditions |
---|---|
Catalyst Type | Acid catalysts (e.g., sulfuric acid) |
Temperature | 90 °C |
Reaction Time | 1-3 hours |
Glycerol to Acetone Ratio | 1:10 |
1. Biodiesel Applications
Solketal acetate has been identified as a promising additive for biodiesel. It significantly improves biodiesel viscosity and stability, which are critical for engine performance. Research indicates that the incorporation of solketal acetate into biodiesel formulations enhances fuel properties:
- Viscosity Improvement : It increases the viscosity index, making biodiesel more suitable for cold weather conditions.
- Combustion Characteristics : Studies have shown that solketal acetate contributes to better combustion efficiency and reduced emissions when blended with traditional biodiesel fuels .
2. Nutritional Aspects
In animal nutrition, solketal acetate derived from crude glycerol has demonstrated potential as an energy source. Research indicates that it can be effectively metabolized by non-ruminant animals:
- Digestible Energy (DE) : Values for crude glycerol samples containing solketal acetate ranged from 14.9 to 15.3 MJ/kg.
- Metabolizable Energy (ME) : Reported ME values for broilers and laying hens were approximately 15.9 MJ/kg, indicating efficient utilization in animal diets .
Case Study 1: Biodiesel Performance Enhancement
A study conducted on biodiesel blends containing solketal acetate revealed significant improvements in cold flow properties compared to standard biodiesel blends. The addition of solketal acetate resulted in:
- A reduction in cloud point by approximately 5 °C.
- Enhanced oxidative stability by extending the induction period by over 20 hours.
These findings suggest that solketal acetate can effectively modify biodiesel properties to meet stringent performance standards.
Case Study 2: Animal Feed Trials
In trials involving broiler chickens, diets supplemented with crude glycerol containing solketal acetate showed improved weight gain and feed conversion ratios compared to control groups:
Properties
IUPAC Name |
(2,2-dimethyl-1,3-dioxolan-4-yl)methyl acetate | |
---|---|---|
Source | PubChem | |
URL | https://pubchem.ncbi.nlm.nih.gov | |
Description | Data deposited in or computed by PubChem | |
InChI |
InChI=1S/C8H14O4/c1-6(9)10-4-7-5-11-8(2,3)12-7/h7H,4-5H2,1-3H3 | |
Source | PubChem | |
URL | https://pubchem.ncbi.nlm.nih.gov | |
Description | Data deposited in or computed by PubChem | |
InChI Key |
CKCWECNJFXXSQU-UHFFFAOYSA-N | |
Source | PubChem | |
URL | https://pubchem.ncbi.nlm.nih.gov | |
Description | Data deposited in or computed by PubChem | |
Canonical SMILES |
CC(=O)OCC1COC(O1)(C)C | |
Source | PubChem | |
URL | https://pubchem.ncbi.nlm.nih.gov | |
Description | Data deposited in or computed by PubChem | |
Molecular Formula |
C8H14O4 | |
Source | PubChem | |
URL | https://pubchem.ncbi.nlm.nih.gov | |
Description | Data deposited in or computed by PubChem | |
DSSTOX Substance ID |
DTXSID70291206 | |
Record name | (2,2-Dimethyl-1,3-dioxolan-4-yl)methyl acetate | |
Source | EPA DSSTox | |
URL | https://comptox.epa.gov/dashboard/DTXSID70291206 | |
Description | DSSTox provides a high quality public chemistry resource for supporting improved predictive toxicology. | |
Molecular Weight |
174.19 g/mol | |
Source | PubChem | |
URL | https://pubchem.ncbi.nlm.nih.gov | |
Description | Data deposited in or computed by PubChem | |
CAS No. |
14739-11-8 | |
Record name | 1, 2,2-dimethyl-, acetate | |
Source | DTP/NCI | |
URL | https://dtp.cancer.gov/dtpstandard/servlet/dwindex?searchtype=NSC&outputformat=html&searchlist=74009 | |
Description | The NCI Development Therapeutics Program (DTP) provides services and resources to the academic and private-sector research communities worldwide to facilitate the discovery and development of new cancer therapeutic agents. | |
Explanation | Unless otherwise indicated, all text within NCI products is free of copyright and may be reused without our permission. Credit the National Cancer Institute as the source. | |
Record name | (2,2-Dimethyl-1,3-dioxolan-4-yl)methyl acetate | |
Source | EPA DSSTox | |
URL | https://comptox.epa.gov/dashboard/DTXSID70291206 | |
Description | DSSTox provides a high quality public chemistry resource for supporting improved predictive toxicology. | |
Synthesis routes and methods I
Procedure details
Synthesis routes and methods II
Procedure details
Synthesis routes and methods III
Procedure details
Retrosynthesis Analysis
AI-Powered Synthesis Planning: Our tool employs the Template_relevance Pistachio, Template_relevance Bkms_metabolic, Template_relevance Pistachio_ringbreaker, Template_relevance Reaxys, Template_relevance Reaxys_biocatalysis model, leveraging a vast database of chemical reactions to predict feasible synthetic routes.
One-Step Synthesis Focus: Specifically designed for one-step synthesis, it provides concise and direct routes for your target compounds, streamlining the synthesis process.
Accurate Predictions: Utilizing the extensive PISTACHIO, BKMS_METABOLIC, PISTACHIO_RINGBREAKER, REAXYS, REAXYS_BIOCATALYSIS database, our tool offers high-accuracy predictions, reflecting the latest in chemical research and data.
Strategy Settings
Precursor scoring | Relevance Heuristic |
---|---|
Min. plausibility | 0.01 |
Model | Template_relevance |
Template Set | Pistachio/Bkms_metabolic/Pistachio_ringbreaker/Reaxys/Reaxys_biocatalysis |
Top-N result to add to graph | 6 |
Feasible Synthetic Routes
Disclaimer and Information on In-Vitro Research Products
Please be aware that all articles and product information presented on BenchChem are intended solely for informational purposes. The products available for purchase on BenchChem are specifically designed for in-vitro studies, which are conducted outside of living organisms. In-vitro studies, derived from the Latin term "in glass," involve experiments performed in controlled laboratory settings using cells or tissues. It is important to note that these products are not categorized as medicines or drugs, and they have not received approval from the FDA for the prevention, treatment, or cure of any medical condition, ailment, or disease. We must emphasize that any form of bodily introduction of these products into humans or animals is strictly prohibited by law. It is essential to adhere to these guidelines to ensure compliance with legal and ethical standards in research and experimentation.