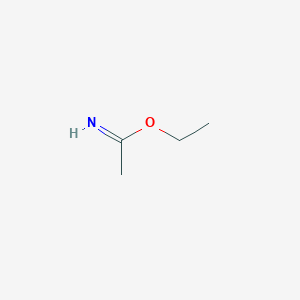
Ethyl acetimidate
Overview
Description
Ethyl acetimidate, also known as ethyl ethanimidate, is an organic compound with the molecular formula C4H9NO. It is commonly used in organic synthesis and chemical research due to its reactivity and versatility. The compound is typically found as its hydrochloride salt, this compound hydrochloride, which is a white crystalline powder that is soluble in water .
Preparation Methods
Synthetic Routes and Reaction Conditions: Ethyl acetimidate can be synthesized through the reaction of ethyl alcohol and acetonitrile in the presence of hydrogen chloride gas. The reaction is typically carried out in a three-necked flask under cryogenic conditions (below 5°C) to ensure high yield and purity . The process involves passing dry hydrogen chloride gas through the mixture of ethyl alcohol and acetonitrile, followed by heating to complete the reaction.
Industrial Production Methods: In industrial settings, the production of this compound hydrochloride follows a similar synthetic route but on a larger scale. The reaction conditions are carefully controlled to maintain the temperature and ensure the efficient conversion of reactants to the desired product. The final product is then purified and crystallized to obtain high-purity this compound hydrochloride .
Chemical Reactions Analysis
Types of Reactions: Ethyl acetimidate undergoes various chemical reactions, including:
Substitution Reactions: It can react with nucleophiles to form substituted products.
Hydrolysis: In the presence of water, this compound can hydrolyze to form ethyl acetate and ammonia.
Condensation Reactions: It can participate in condensation reactions with carbonyl compounds to form imines.
Common Reagents and Conditions:
Nucleophiles: Such as amines and alcohols.
Acidic or Basic Conditions: Depending on the desired reaction pathway.
Solvents: Common solvents include dichloromethane and acetonitrile.
Major Products:
Substituted Imidates: Formed through substitution reactions.
Ethyl Acetate and Ammonia: Formed through hydrolysis.
Scientific Research Applications
Ethyl acetimidate is widely used in scientific research due to its reactivity and versatility. Some of its applications include:
Chemical Synthesis: Used as a reagent in the synthesis of various organic compounds.
Biological Research: Employed in the modification of proteins and enzymes, such as the amidination of carbonic anhydrase.
Medicinal Chemistry: Utilized in the synthesis of pharmaceutical intermediates and active compounds.
Industrial Applications: Used in the production of specialty chemicals and materials.
Mechanism of Action
The mechanism of action of ethyl acetimidate involves its reactivity with nucleophiles. The compound can form covalent bonds with nucleophilic sites on proteins, enzymes, and other molecules, leading to the formation of stable imidate derivatives. This reactivity is exploited in various chemical and biological applications, such as protein modification and enzyme inhibition .
Comparison with Similar Compounds
Ethyl acetimidate is similar to other imidate compounds, such as mthis compound and propyl acetimidate. it is unique in its reactivity and solubility properties, making it particularly useful in specific applications. Some similar compounds include:
Mthis compound: Similar reactivity but different solubility properties.
Propyl Acetimidate: Similar reactivity but different chain length and physical properties.
This compound stands out due to its optimal balance of reactivity and solubility, making it a preferred choice in many chemical and biological applications .
Properties
IUPAC Name |
ethyl ethanimidate | |
---|---|---|
Source | PubChem | |
URL | https://pubchem.ncbi.nlm.nih.gov | |
Description | Data deposited in or computed by PubChem | |
InChI |
InChI=1S/C4H9NO/c1-3-6-4(2)5/h5H,3H2,1-2H3 | |
Source | PubChem | |
URL | https://pubchem.ncbi.nlm.nih.gov | |
Description | Data deposited in or computed by PubChem | |
InChI Key |
JMIAPORGEDIDLT-UHFFFAOYSA-N | |
Source | PubChem | |
URL | https://pubchem.ncbi.nlm.nih.gov | |
Description | Data deposited in or computed by PubChem | |
Canonical SMILES |
CCOC(=N)C | |
Source | PubChem | |
URL | https://pubchem.ncbi.nlm.nih.gov | |
Description | Data deposited in or computed by PubChem | |
Molecular Formula |
C4H9NO | |
Source | PubChem | |
URL | https://pubchem.ncbi.nlm.nih.gov | |
Description | Data deposited in or computed by PubChem | |
Related CAS |
2208-07-3 (hydrochloride) | |
Record name | Ethyl acetimidate | |
Source | ChemIDplus | |
URL | https://pubchem.ncbi.nlm.nih.gov/substance/?source=chemidplus&sourceid=0001000846 | |
Description | ChemIDplus is a free, web search system that provides access to the structure and nomenclature authority files used for the identification of chemical substances cited in National Library of Medicine (NLM) databases, including the TOXNET system. | |
DSSTOX Substance ID |
DTXSID4061381 | |
Record name | Ethanimidic acid, ethyl ester | |
Source | EPA DSSTox | |
URL | https://comptox.epa.gov/dashboard/DTXSID4061381 | |
Description | DSSTox provides a high quality public chemistry resource for supporting improved predictive toxicology. | |
Molecular Weight |
87.12 g/mol | |
Source | PubChem | |
URL | https://pubchem.ncbi.nlm.nih.gov | |
Description | Data deposited in or computed by PubChem | |
CAS No. |
1000-84-6 | |
Record name | Ethyl acetimidate | |
Source | CAS Common Chemistry | |
URL | https://commonchemistry.cas.org/detail?cas_rn=1000-84-6 | |
Description | CAS Common Chemistry is an open community resource for accessing chemical information. Nearly 500,000 chemical substances from CAS REGISTRY cover areas of community interest, including common and frequently regulated chemicals, and those relevant to high school and undergraduate chemistry classes. This chemical information, curated by our expert scientists, is provided in alignment with our mission as a division of the American Chemical Society. | |
Explanation | The data from CAS Common Chemistry is provided under a CC-BY-NC 4.0 license, unless otherwise stated. | |
Record name | Ethyl acetimidate | |
Source | ChemIDplus | |
URL | https://pubchem.ncbi.nlm.nih.gov/substance/?source=chemidplus&sourceid=0001000846 | |
Description | ChemIDplus is a free, web search system that provides access to the structure and nomenclature authority files used for the identification of chemical substances cited in National Library of Medicine (NLM) databases, including the TOXNET system. | |
Record name | Ethanimidic acid, ethyl ester | |
Source | EPA Chemicals under the TSCA | |
URL | https://www.epa.gov/chemicals-under-tsca | |
Description | EPA Chemicals under the Toxic Substances Control Act (TSCA) collection contains information on chemicals and their regulations under TSCA, including non-confidential content from the TSCA Chemical Substance Inventory and Chemical Data Reporting. | |
Record name | Ethanimidic acid, ethyl ester | |
Source | EPA DSSTox | |
URL | https://comptox.epa.gov/dashboard/DTXSID4061381 | |
Description | DSSTox provides a high quality public chemistry resource for supporting improved predictive toxicology. | |
Record name | Ethyl acetimidate | |
Source | European Chemicals Agency (ECHA) | |
URL | https://echa.europa.eu/substance-information/-/substanceinfo/100.012.433 | |
Description | The European Chemicals Agency (ECHA) is an agency of the European Union which is the driving force among regulatory authorities in implementing the EU's groundbreaking chemicals legislation for the benefit of human health and the environment as well as for innovation and competitiveness. | |
Explanation | Use of the information, documents and data from the ECHA website is subject to the terms and conditions of this Legal Notice, and subject to other binding limitations provided for under applicable law, the information, documents and data made available on the ECHA website may be reproduced, distributed and/or used, totally or in part, for non-commercial purposes provided that ECHA is acknowledged as the source: "Source: European Chemicals Agency, http://echa.europa.eu/". Such acknowledgement must be included in each copy of the material. ECHA permits and encourages organisations and individuals to create links to the ECHA website under the following cumulative conditions: Links can only be made to webpages that provide a link to the Legal Notice page. | |
Retrosynthesis Analysis
AI-Powered Synthesis Planning: Our tool employs the Template_relevance Pistachio, Template_relevance Bkms_metabolic, Template_relevance Pistachio_ringbreaker, Template_relevance Reaxys, Template_relevance Reaxys_biocatalysis model, leveraging a vast database of chemical reactions to predict feasible synthetic routes.
One-Step Synthesis Focus: Specifically designed for one-step synthesis, it provides concise and direct routes for your target compounds, streamlining the synthesis process.
Accurate Predictions: Utilizing the extensive PISTACHIO, BKMS_METABOLIC, PISTACHIO_RINGBREAKER, REAXYS, REAXYS_BIOCATALYSIS database, our tool offers high-accuracy predictions, reflecting the latest in chemical research and data.
Strategy Settings
Precursor scoring | Relevance Heuristic |
---|---|
Min. plausibility | 0.01 |
Model | Template_relevance |
Template Set | Pistachio/Bkms_metabolic/Pistachio_ringbreaker/Reaxys/Reaxys_biocatalysis |
Top-N result to add to graph | 6 |
Feasible Synthetic Routes
Disclaimer and Information on In-Vitro Research Products
Please be aware that all articles and product information presented on BenchChem are intended solely for informational purposes. The products available for purchase on BenchChem are specifically designed for in-vitro studies, which are conducted outside of living organisms. In-vitro studies, derived from the Latin term "in glass," involve experiments performed in controlled laboratory settings using cells or tissues. It is important to note that these products are not categorized as medicines or drugs, and they have not received approval from the FDA for the prevention, treatment, or cure of any medical condition, ailment, or disease. We must emphasize that any form of bodily introduction of these products into humans or animals is strictly prohibited by law. It is essential to adhere to these guidelines to ensure compliance with legal and ethical standards in research and experimentation.