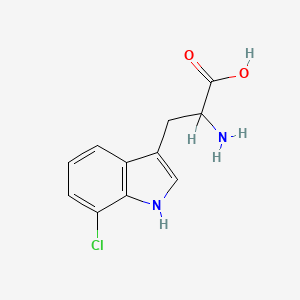
7-Chlorotryptophan
Overview
Description
7-chloro-L-tryptophan is an L-tryptophan derivative having a chloro substituent at the 7-position. It is a L-tryptophan derivative, a 7-chlorotryptophan and a non-proteinogenic L-alpha-amino acid. It is an enantiomer of a 7-chloro-D-tryptophan. It is a tautomer of a 7-chloro-L-tryptophan zwitterion.
Mechanism of Action
Target of Action
7-Chloro-DL-Tryptophan, also known as 7-Chlorotryptophan, primarily targets the enzymes involved in tryptophan metabolism . These enzymes play a crucial role in the conversion of tryptophan into various bioactive molecules .
Mode of Action
The compound interacts with its targets by undergoing a halogenation reaction, which is catalyzed by RebF/RebH to generate 7-chloro-tryptophan . Following this, RebO, a FAD-dependent L-Trp oxidase, converts 7-chloro-tryptophan to 7-chloroindole-3-pyruvic acid imine by releasing hydrogen peroxide .
Biochemical Pathways
7-Chloro-DL-Tryptophan affects the tryptophan metabolism pathway . This pathway is complex and results in the production of many bioactive molecules that act in various organs through different action mechanisms . The compound’s interaction with the enzymes involved in tryptophan metabolism can influence these downstream effects .
Result of Action
Given its role in tryptophan metabolism, it may influence the production of bioactive molecules derived from tryptophan . These molecules can have various effects on physiological processes, including gastrointestinal functions, immunity, metabolism, and the nervous system .
Biochemical Analysis
Biochemical Properties
7-Chloro-DL-Tryptophan is involved in various biochemical reactions. It is produced through a combination of L-amino acid deaminase (L-AAD) and halogenase, which catalyze the conversion of an L-amino acid to halide and an α-keto acid . The optimal activity ratio of L-AAD and halogenase is set between 1:50 and 1:60 .
Cellular Effects
The effects of 7-Chloro-DL-Tryptophan on various types of cells and cellular processes are significant. It has been shown to influence cell function, including impacts on cell signaling pathways, gene expression, and cellular metabolism . For instance, it has been reported that 7-Chloro-DL-Tryptophan can improve blood pressure, blood glucose, and insulin levels .
Molecular Mechanism
The molecular mechanism of 7-Chloro-DL-Tryptophan involves its interactions with biomolecules and changes in gene expression. It is known to interact with multiple downstream enzymes, accessing unexplored regions of metabolism
Temporal Effects in Laboratory Settings
In laboratory settings, the effects of 7-Chloro-DL-Tryptophan change over time. Within 6 hours, significant amounts of 7-Chloro-DL-Tryptophan and indole pyruvic acid (IPA) were synthesized in a selected mono-amino acid system . The product’s stability, degradation, and long-term effects on cellular function observed in in vitro or in vivo studies are still being explored.
Metabolic Pathways
7-Chloro-DL-Tryptophan is involved in the kynurenine pathway of tryptophan metabolism . This pathway is involved in inflammation, immune responses, and excitatory neurotransmission . The key regulatory enzymes in this pathway can be targeted for treating several diseases .
Transport and Distribution
It is known that it is produced in Escherichia coli from glucose
Subcellular Localization
It is known that it is produced in Escherichia coli from glucose
Properties
IUPAC Name |
2-amino-3-(7-chloro-1H-indol-3-yl)propanoic acid | |
---|---|---|
Source | PubChem | |
URL | https://pubchem.ncbi.nlm.nih.gov | |
Description | Data deposited in or computed by PubChem | |
InChI |
InChI=1S/C11H11ClN2O2/c12-8-3-1-2-7-6(5-14-10(7)8)4-9(13)11(15)16/h1-3,5,9,14H,4,13H2,(H,15,16) | |
Source | PubChem | |
URL | https://pubchem.ncbi.nlm.nih.gov | |
Description | Data deposited in or computed by PubChem | |
InChI Key |
DMQFGLHRDFQKNR-UHFFFAOYSA-N | |
Source | PubChem | |
URL | https://pubchem.ncbi.nlm.nih.gov | |
Description | Data deposited in or computed by PubChem | |
Canonical SMILES |
C1=CC2=C(C(=C1)Cl)NC=C2CC(C(=O)O)N | |
Source | PubChem | |
URL | https://pubchem.ncbi.nlm.nih.gov | |
Description | Data deposited in or computed by PubChem | |
Molecular Formula |
C11H11ClN2O2 | |
Source | PubChem | |
URL | https://pubchem.ncbi.nlm.nih.gov | |
Description | Data deposited in or computed by PubChem | |
Molecular Weight |
238.67 g/mol | |
Source | PubChem | |
URL | https://pubchem.ncbi.nlm.nih.gov | |
Description | Data deposited in or computed by PubChem | |
CAS No. |
153-97-9 | |
Record name | 7-Chlorotryptophan | |
Source | CAS Common Chemistry | |
URL | https://commonchemistry.cas.org/detail?cas_rn=153-97-9 | |
Description | CAS Common Chemistry is an open community resource for accessing chemical information. Nearly 500,000 chemical substances from CAS REGISTRY cover areas of community interest, including common and frequently regulated chemicals, and those relevant to high school and undergraduate chemistry classes. This chemical information, curated by our expert scientists, is provided in alignment with our mission as a division of the American Chemical Society. | |
Explanation | The data from CAS Common Chemistry is provided under a CC-BY-NC 4.0 license, unless otherwise stated. | |
Retrosynthesis Analysis
AI-Powered Synthesis Planning: Our tool employs the Template_relevance Pistachio, Template_relevance Bkms_metabolic, Template_relevance Pistachio_ringbreaker, Template_relevance Reaxys, Template_relevance Reaxys_biocatalysis model, leveraging a vast database of chemical reactions to predict feasible synthetic routes.
One-Step Synthesis Focus: Specifically designed for one-step synthesis, it provides concise and direct routes for your target compounds, streamlining the synthesis process.
Accurate Predictions: Utilizing the extensive PISTACHIO, BKMS_METABOLIC, PISTACHIO_RINGBREAKER, REAXYS, REAXYS_BIOCATALYSIS database, our tool offers high-accuracy predictions, reflecting the latest in chemical research and data.
Strategy Settings
Precursor scoring | Relevance Heuristic |
---|---|
Min. plausibility | 0.01 |
Model | Template_relevance |
Template Set | Pistachio/Bkms_metabolic/Pistachio_ringbreaker/Reaxys/Reaxys_biocatalysis |
Top-N result to add to graph | 6 |
Feasible Synthetic Routes
Q1: What is the biological role of 7-chlorotryptophan and how is it involved in the biosynthesis of secondary metabolites?
A1: this compound is not a naturally occurring amino acid, but rather a halogenated derivative of tryptophan. It serves as a key intermediate in the biosynthesis of various bioactive secondary metabolites, including the antifungal agent pyrrolnitrin [** ]. The incorporation of the chlorine atom at the 7-position of the indole ring is often crucial for the biological activity of these compounds.
Q2: Which enzymes are involved in the production of this compound and how do their activities differ?
A2: this compound is produced through the enzymatic chlorination of L-tryptophan. This reaction is catalyzed by flavin-dependent halogenases, specifically two-component systems involving a flavin reductase (e.g., RebF) and a halogenase (e.g., RebH) . The reductase provides the reduced FADH2 required for the halogenase to activate chloride ions and subsequently halogenate the substrate. Variations in substrate specificity and regioselectivity exist among different halogenases. For example, while RebH primarily produces this compound, other halogenases like ThdN from Streptomyces albogriseolus exhibit a preference for 6-chlorotryptophan and can also utilize brominated tryptophan derivatives .
Q3: How does the presence of this compound affect the metabolic flux in organisms that produce it?
A3: In organisms engineered to produce this compound, its accumulation can create a metabolic bottleneck. For instance, in periwinkle plants expressing the RebH halogenase, high levels of this compound were observed . This accumulation is attributed to the low efficiency of tryptophan decarboxylase, a key enzyme in alkaloid biosynthesis, to utilize this compound as a substrate compared to its natural substrate, tryptophan.
Q4: What is the mechanism of action of flavin-dependent halogenases in producing this compound?
A4: Flavin-dependent halogenases utilize a multistep mechanism to chlorinate tryptophan . First, the flavin reductase reduces FAD to FADH2, which subsequently reacts with molecular oxygen to form a FAD(C4a)-OOH intermediate. This intermediate reacts with chloride ions to generate hypochlorous acid (HOCl), a powerful chlorinating agent. While initially believed to directly chlorinate tryptophan, evidence suggests the involvement of a long-lived chlorinating intermediate. This intermediate, proposed to be a lysine chloramine formed by the reaction of HOCl with an active site lysine residue (Lys79 in RebH), then regioselectively chlorinates tryptophan to yield this compound .
Q5: Can the substrate specificity of flavin-dependent halogenases be altered to produce other halogenated tryptophan derivatives?
A5: Yes, research has shown that modifying the active site of flavin-dependent halogenases can alter their substrate specificity. For instance, the RebH Y455W mutant exhibits a shift in substrate preference from tryptophan to tryptamine, leading to the production of halogenated tryptamine alkaloids in periwinkle plants . This highlights the potential for protein engineering to expand the repertoire of halogenated tryptophan derivatives accessible through enzymatic synthesis.
Q6: What are the potential applications of this compound in drug discovery and development?
A6: this compound holds promise as a building block for generating novel drug leads. Its presence as a precursor to various bioactive natural products suggests that it could be used to create libraries of structurally diverse compounds with potentially valuable pharmacological properties. Additionally, understanding the enzymatic pathways involved in its biosynthesis can inform the development of biocatalytic approaches for the sustainable production of pharmaceuticals and other fine chemicals .
Q7: Beyond pyrrolnitrin, what other secondary metabolites are derived from this compound?
A7: this compound is a precursor to various halogenated tryptamine alkaloids . These alkaloids exhibit a range of biological activities and include compounds like 12-chloro-19,20-dihydroakuammicine, identified in periwinkle plants engineered with the RebH Y455W mutant.
Q8: How is this compound metabolized by microorganisms?
A8: Certain Pseudomonas species, such as Pseudomonas pyrrocinia and Pseudomonas aureofaciens, can metabolize this compound through a pathway involving transamination, decarboxylation, and oxidation reactions . This pathway leads to the formation of various metabolites including indole-3-carboxylic acid, indole-3-acetic acid, and their respective chlorinated analogs.
Q9: What are the implications of this compound metabolism for the bioremediation of environmental pollutants?
A9: Understanding the microbial degradation pathways of this compound and its related compounds is crucial for developing bioremediation strategies. These strategies aim to utilize microorganisms to remove halogenated organic compounds, often considered environmental pollutants, from contaminated soil and water resources.
Q10: How can tryptophan decarboxylase be utilized for the production of novel tryptamine derivatives from halogenated tryptophans?
A10: Tryptophan decarboxylases, like BaTDC from Bacillus atrophaeus C89, exhibit broad substrate specificity and can catalyze the decarboxylation of various tryptophan derivatives, including halogenated tryptophans . This property allows for the enzymatic synthesis of novel tryptamine analogs, which are valuable building blocks for pharmaceutical research and drug development.
Q11: What analytical techniques are used to detect and quantify this compound and related metabolites?
A12: Various analytical techniques are employed to study this compound and related metabolites. High-performance liquid chromatography (HPLC) coupled with ultraviolet (UV) or mass spectrometry (MS) detection is commonly used to separate and quantify these compounds in complex mixtures . Spectroscopic techniques, such as nuclear magnetic resonance (NMR) spectroscopy, are essential for structural characterization.
Disclaimer and Information on In-Vitro Research Products
Please be aware that all articles and product information presented on BenchChem are intended solely for informational purposes. The products available for purchase on BenchChem are specifically designed for in-vitro studies, which are conducted outside of living organisms. In-vitro studies, derived from the Latin term "in glass," involve experiments performed in controlled laboratory settings using cells or tissues. It is important to note that these products are not categorized as medicines or drugs, and they have not received approval from the FDA for the prevention, treatment, or cure of any medical condition, ailment, or disease. We must emphasize that any form of bodily introduction of these products into humans or animals is strictly prohibited by law. It is essential to adhere to these guidelines to ensure compliance with legal and ethical standards in research and experimentation.