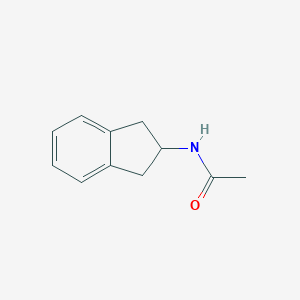
N-(2,3-Dihydro-1H-inden-2-yl)acetamide
Overview
Description
N-(2,3-Dihydro-1H-inden-2-yl)acetamide, also known as indenylacetamide, is a compound that has gained significant attention in the scientific community due to its potential applications in various fields. This compound has been synthesized using different methods, and its mechanism of action, biochemical and physiological effects, and future directions have been extensively studied.
Scientific Research Applications
Anticancer Activity: Synthesis of 1H-inden-1-one substituted (Heteroaryl)Acetamide derivatives has shown promising anticancer activities. Certain compounds within this class exhibited significant growth inhibition against leukemia, non-small cell lung cancer, and breast cancer cell lines (Karaburun et al., 2018).
Cytotoxic Agents: Novel 2-(2,3-dioxo-2,3-dihydro-1H-indol-1-yl)-N-phenylacetamide derivatives synthesized from isatin showed cytotoxic activity against breast cancer cell lines, indicating their potential as anticancer agents (Modi et al., 2011).
Analgesic Activity: Acetamide derivatives were synthesized and tested for their analgesic properties. These compounds showed significant analgesic effects in various tests, indicating their potential for pain relief (Kaplancıklı et al., 2012).
Anti-Diabetic Agents: A study on 2-[2,3-dihydro-1,4-benzodioxin-6-yl(phenylsulfonyl)amino]-N-(un/substituted-phenyl)acetamides showed weak to moderate inhibitory activities against α-glucosidase enzyme, suggesting their potential as anti-diabetic agents (Abbasi et al., 2023).
Antimicrobial Activity: N-substituted benzimidazole acetamide derivatives were synthesized and evaluated for their activity against Methicillin Resistant Staphylococcus aureus (MRSA), showing significant potent antibacterial activity (Chaudhari et al., 2020).
Antioxidant Properties: Synthesis of N-(substituted phenyl)-2-(3-(hydroxyimino) methyl)-1H-indol-1-yl) acetamide derivatives and their evaluation for antioxidant activity showed that these compounds possess significant antioxidant properties (Gopi & Dhanaraju, 2020).
Mechanism of Action
Target of Action
It’s known that indole derivatives, which this compound is a part of, bind with high affinity to multiple receptors . These receptors are often involved in a variety of biological activities, including antiviral, anti-inflammatory, anticancer, anti-HIV, antioxidant, antimicrobial, antitubercular, antidiabetic, antimalarial, and anticholinesterase activities .
Mode of Action
Indole derivatives are known to interact with their targets in a way that modulates various biological activities .
Biochemical Pathways
Indole derivatives are known to influence a variety of biochemical pathways due to their broad-spectrum biological activities .
Pharmacokinetics
The compound has a molecular weight of 17523 Da , which is within the range generally favorable for oral bioavailability in drug design.
Result of Action
Indole derivatives are known to have a wide range of biological effects, including antiviral, anti-inflammatory, anticancer, anti-hiv, antioxidant, antimicrobial, antitubercular, antidiabetic, antimalarial, and anticholinesterase activities .
Properties
IUPAC Name |
N-(2,3-dihydro-1H-inden-2-yl)acetamide | |
---|---|---|
Source | PubChem | |
URL | https://pubchem.ncbi.nlm.nih.gov | |
Description | Data deposited in or computed by PubChem | |
InChI |
InChI=1S/C11H13NO/c1-8(13)12-11-6-9-4-2-3-5-10(9)7-11/h2-5,11H,6-7H2,1H3,(H,12,13) | |
Source | PubChem | |
URL | https://pubchem.ncbi.nlm.nih.gov | |
Description | Data deposited in or computed by PubChem | |
InChI Key |
AVBOEYUWFJGVRI-UHFFFAOYSA-N | |
Source | PubChem | |
URL | https://pubchem.ncbi.nlm.nih.gov | |
Description | Data deposited in or computed by PubChem | |
Canonical SMILES |
CC(=O)NC1CC2=CC=CC=C2C1 | |
Source | PubChem | |
URL | https://pubchem.ncbi.nlm.nih.gov | |
Description | Data deposited in or computed by PubChem | |
Molecular Formula |
C11H13NO | |
Source | PubChem | |
URL | https://pubchem.ncbi.nlm.nih.gov | |
Description | Data deposited in or computed by PubChem | |
DSSTOX Substance ID |
DTXSID70498854 | |
Record name | N-(2,3-Dihydro-1H-inden-2-yl)acetamide | |
Source | EPA DSSTox | |
URL | https://comptox.epa.gov/dashboard/DTXSID70498854 | |
Description | DSSTox provides a high quality public chemistry resource for supporting improved predictive toxicology. | |
Molecular Weight |
175.23 g/mol | |
Source | PubChem | |
URL | https://pubchem.ncbi.nlm.nih.gov | |
Description | Data deposited in or computed by PubChem | |
CAS No. |
13935-80-3 | |
Record name | N-(2,3-Dihydro-1H-inden-2-yl)acetamide | |
Source | CAS Common Chemistry | |
URL | https://commonchemistry.cas.org/detail?cas_rn=13935-80-3 | |
Description | CAS Common Chemistry is an open community resource for accessing chemical information. Nearly 500,000 chemical substances from CAS REGISTRY cover areas of community interest, including common and frequently regulated chemicals, and those relevant to high school and undergraduate chemistry classes. This chemical information, curated by our expert scientists, is provided in alignment with our mission as a division of the American Chemical Society. | |
Explanation | The data from CAS Common Chemistry is provided under a CC-BY-NC 4.0 license, unless otherwise stated. | |
Record name | N-(2,3-Dihydro-1H-inden-2-yl)acetamide | |
Source | EPA DSSTox | |
URL | https://comptox.epa.gov/dashboard/DTXSID70498854 | |
Description | DSSTox provides a high quality public chemistry resource for supporting improved predictive toxicology. | |
Synthesis routes and methods I
Procedure details
Synthesis routes and methods II
Procedure details
Synthesis routes and methods III
Procedure details
Synthesis routes and methods IV
Procedure details
Synthesis routes and methods V
Procedure details
Retrosynthesis Analysis
AI-Powered Synthesis Planning: Our tool employs the Template_relevance Pistachio, Template_relevance Bkms_metabolic, Template_relevance Pistachio_ringbreaker, Template_relevance Reaxys, Template_relevance Reaxys_biocatalysis model, leveraging a vast database of chemical reactions to predict feasible synthetic routes.
One-Step Synthesis Focus: Specifically designed for one-step synthesis, it provides concise and direct routes for your target compounds, streamlining the synthesis process.
Accurate Predictions: Utilizing the extensive PISTACHIO, BKMS_METABOLIC, PISTACHIO_RINGBREAKER, REAXYS, REAXYS_BIOCATALYSIS database, our tool offers high-accuracy predictions, reflecting the latest in chemical research and data.
Strategy Settings
Precursor scoring | Relevance Heuristic |
---|---|
Min. plausibility | 0.01 |
Model | Template_relevance |
Template Set | Pistachio/Bkms_metabolic/Pistachio_ringbreaker/Reaxys/Reaxys_biocatalysis |
Top-N result to add to graph | 6 |
Feasible Synthetic Routes
Disclaimer and Information on In-Vitro Research Products
Please be aware that all articles and product information presented on BenchChem are intended solely for informational purposes. The products available for purchase on BenchChem are specifically designed for in-vitro studies, which are conducted outside of living organisms. In-vitro studies, derived from the Latin term "in glass," involve experiments performed in controlled laboratory settings using cells or tissues. It is important to note that these products are not categorized as medicines or drugs, and they have not received approval from the FDA for the prevention, treatment, or cure of any medical condition, ailment, or disease. We must emphasize that any form of bodily introduction of these products into humans or animals is strictly prohibited by law. It is essential to adhere to these guidelines to ensure compliance with legal and ethical standards in research and experimentation.